On the Origin of the Warburg Effect in Cancer Cells: Controlling Cancer as a Metabolic Disease
Download
Abstract
Background: Human cells may switch metabolism from aerobic to anaerobic or vice versa depending on cellular conditions. The use of anaerobic cellular respiration is especially common in cells with metabolic disorders such as cancer cells. However, despite the fact that metabolic alteration in cancer cells is well-established, its cause is still not well understood. Objective: The purpose of this study is to address the origin of abnormal behavior and metabolic changes in cancer cells to better understand the processes that are involved in the formation and spread of cancer.
Methods: This paper reviews and explains key concepts related to the evolutionary origin of key metabolic pathways in cancer cells, considering the behavioral similarities between cancer cells and ancient unicellular organisms. The evaluations help better understand the Warburg effect and the related cancer control strategies.
Results: The risk of cancer may be reduced by creating suitable and optimal conditions at the cellular level, which can increase the chance of cell survival in the event of cellular stress. This may be achieved through dietary and lifestyle modifications, such as adopting a balanced natural diet that meets cellular needs in a way that leads to cancer control.
Conclusions: Understanding the biological origin and causes of cancer initiation and development is essential for the metabolic control of cancer as well as for improving therapeutic strategies.
Introduction
The first living cells were single-celled organisms that lived in the harsh conditions of the ancient Earth with little atmospheric oxygen about 3-4 billion years ago. These single-celled organisms, common ancestor of all known forms of life on earth, respired anaerobically in absence of oxygen and used sulfur oxidation using hydrogen sulfide (H2S) as a cellular energy source [1-4]. From about 600 to 500 million years ago, when the oxygen in the Earth’s atmosphere increased significantly, as well as the ozone layer gradually formed that protected the planet from harmful cosmic and ultraviolet rays, the conditions for life on Earth changed significantly. The rise of oxygen level was toxic to anaerobic systems, and much of the anaerobic life died out, triggering a mass extinction event. This ended the use of H2S as a common energy source, and most H2S-dependent organisms died [4-6]. After the significant increase of atmospheric oxygen, only the fittest cells that could adapt to the altered conditions could survive. The survived cells managed to utilize the rich potential of oxygen in respiration, and soon, oxygen became essential for metabolic activities in oxygenated environments. Because oxygen is ideal for generating energy from nutrients in cells, aerobic life in oxygenated environments evolved significantly faster and more efficiently than anaerobic life [4,7,8].
To conceptually understand the evolutionary path of the first single cells in the harsh conditions of life on ancient Earth, compare them to a car moving forward on a rocky road with large bumps, using a lower gear to continue with less speed but more power, consuming extra fuel. Similarly, the evolutionary changes following a significant increase in atmospheric oxygen levels can be compared to a car going from a gravel road with large bumps to an asphalt road, which allows the use of a higher gear for faster travel with lower fuel consumption, although the intelligent driver still remembers to downshift if he encounters tough road conditions again.
The aerobic processes that used oxygen as an oxidant to convert nutrients into energy were significantly more efficient at providing cellular energy. Enzymes were also used by the cells to catalyze chemical reactions and accelerate evolution of biochemical reactions. As a result, with passage of time, some larger and more complex living systems were evolved, eventually giving rise to plants, animals, and humans [4, 9-11].
Single celled organisms on ancient earth were self-sufficient and independent, caring only for themselves and greedily seeking nutrients to survive. But in modern multicellular systems, cells used a cooperative approach and became more dependent on other cells, working together to interact with the environment, take up nutrients and water, get rid of wastes, and produce sufficient cellular energy to survive [4,12].
The modern multicellular systems that use aerobic cellular respiration processes in suitable oxygenated environments have their roots in single-celled organisms that respired anaerobically in the harsh living conditions of ancient earth. Hence, modern aerobically respiring cells, when faced with harsh conditions and subjected to cellular stress, may switch metabolism from aerobic to anaerobic (like shifting a car from high gear to low gear), or vice versa, to increase their chances of survival [4,12]. Modern cells typically obey the rules of cooperation, do not move around the body, intelligently control their growth and do not grow further when they encounter other cells to respect their territory, and also divide nutrients fairly to ensure that other organs can also survive. But under stressful conditions and chronic exposure to environmental stressors, cells struggling to survive may undergo some mutations that lead to a return to the behavior of their unicellular ancestors (an evolutionary throwback), following which, they act greedily, take over the body’s resources for their own survival, and disrupt multicellular co-operation, which is a typical behavior that is commonly observed in mutated cancer cells [4, 9,10,12,13].
The cell mutations are not necessarily due to defects, but are the result of metabolic switch and activation of survival mechanisms that cells use to gain greater resistance in harsh living conditions [4,14]. However, under certain conditions, these mutations may lead to the formation of a benign tumor that does not spread to other parts of the body, or, depending on the cellular conditions and accumulation rate of DNA mutations over time, it may form aggressive cancerous malignant tumors that can be life threatening [15-18].
Despite decades of research, the exact origins and causes of behavioral abnormalities in cancer cells have been a long-standing question, and the biological mechanisms by which tumor cells survive and grow remain unclear. Consequently, anticancer strategies for cancer as a metabolic disease are not well understood [4,19]. This article reviews key concepts related to metabolic pathways in cancer cells, focusing on cancer control strategies according to Warburg’s theory of cancer.
The Warburg effect in cancer cells
The mystery of the respiration processes in cancer cells was first revealed by Dr. Otto Heinrich Warburg (1883-1970). Otto Warburg received his first doctoral degree in chemistry in 1906, and earned a second doctorate in medicine in 1911, as well as a degree in physiology in 1913. Otto Warburg received the Nobel Prize in Medicine and Physiology in 1931 for unraveling the oxygen-transferring ferment of respiration [20]. In 1956, Warburg published an article entitled ‘On the origin of cancer cells’ in the journal of Science [21], which was very influential for many scientists, and to date, more than 14,000 scholarly articles have been produced citing this article, and nearly 20,000 articles have discussed Warburg’s work so far, each attempting to interpret it more precisely. Warburg continued to work on fundamental cancer research until his death in 1970 from pulmonary embolism [20,22,23].
Warburg chemically analyzed cancer cells, and based on laboratory measurements and monitoring levels of glucose, acids, and bicarbonates, he experimentally compared oxygen consumption and acid production related to energy metabolism in normal cells and cancer cells. Warburg observed that there are higher levels of lactic acid in the blood vessels that exit the tumor than the arteries that enter the tumor, meaning that, cancer tumors produce significant amounts of lactic acid [20,22,23].
In normal conditions, healthy cells utilize aerobic cellular respiration to produce the energy (ATP) they need [24]:
Glucose (1 C6H12O6) + Oxygen (6 O2) → Energy (32 ATP) + Carbon dioxide (6 CO2) + Water (6 H2O)
But when cellular respiration is impaired, such as in case of oxygen deprivation or hypoxia, a metabolic switch takes place to use anaerobic cellular respiration and fermentation processes, which based on Warburg’s findings (later known as the Warburg effect), may consume large amounts of glucose and produce significant amounts of lactic acid as byproduct that leads to lactic acidosis as follows [4,24]:
Glucose (16 C6H12O6)→ Energy (32 ATP) + Lactic Acid (32 C3H6O3)
According to the Warburg effect, the processes of anaerobic respiration and fermentation, which are energetically inefficient and environmentally acidic and toxic, originally occur in cells lacking oxygen, such as those far from blood vessels, or, in case of hypoxia due to low partial pressure of oxygen, impaired ability of hemoglobin to carry oxygen, or around the cells that consume high amounts of oxygen [20,24,25].
Figure 1 describes the Warburg effect, showing how glucose is converted into energy in normal cells and cancer cells [4,5]. Human cells normally respire aerobically and use glucose as their preferred energy source, most of which is converted to water and carbon dioxide as byproducts of aerobic cellular respiration. But if there is not enough oxygen, the cells produce energy through the processes of anaerobic cellular respiration, where most of the glucose consumed in anaerobically respiring cells is fermented to lactate instead of being oxidized in pathways that require aerobic respiration [4,24].
Figure 1. The Warburg Effect in Normal Cells and Cancer Cells [4,5].
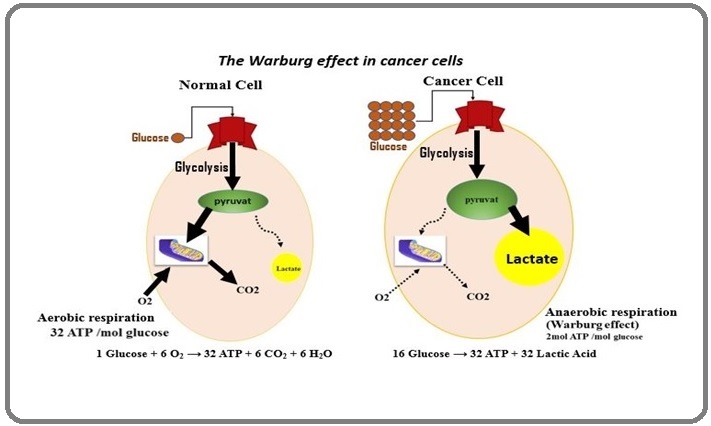
In all types of cellular respiration, the first step in the breakdown of glucose in the cell to extract energy for cellular metabolism is glycolysis, which is anaerobic and does not require oxygen, whether oxygen is present or not (this step is called aerobic glycolysis, which is not what Warburg’s experiments focused on). In the next step, if oxygen is present sufficiently, the pathway will continue on to oxidative phosphorylation, in which, glucose reacts with oxygen in aerobic cellular respiration to produce energy. However, if there is no oxygen, normal cells use anaerobic cellular respiration and fermentation processes, a common starting point of human cancers in hypoxic areas of the body according to the Warburg hypothesis [24,25].
Conceptually, the processes involved in cellular energy production can be compared to starting a car engine and driving. When the car’s engine is to be started, it doesn’t matter if the car is on a gravel road or an asphalt road, in the first step, the car must be started in low gear first. In the next step, if the road is asphalt, driving in high gear can continue. However, if the road is rocky and has big bumps, driving should be continued in low gear. The comparison may help understand why glycolysis, an anaerobic process, is the first step in all cellular respiration processes, both aerobic and anaerobic [4]. That is why in modern aerobically respiring cells evolved from anaerobic life, energy production begins anaerobically, and then proceeds either aerobically or anaerobically, depending on the cellular conditions and availability of oxygen [4]. In potentially hypoxic areas of the body, such as breast tissue in women, or the prostate gland in men, chronic lack of oxygen is a common cause of cancer [25]. An impaired cellular respiration may also be caused by other factors, such as deficiencies in essential nutrients, viruses, infections, or exposure to cellular environmental stressors including acids, chemicals, toxins, and radiation, which in this case, cancer cells grow, multiply, and form tumors even when oxygen is available. When cells are affected by these factors, they use anaerobic cellular respiration processes that produce lactic acid and acidify the cell environment (the primary effect) [4,26,27]. The produced lactic acid itself is also a stressor for cells, leading to inflammation and further cell mutations, and eventually, formation of tumors (the secondary effect) [4,28-32]. This is supported by research studies on prostate cancer, indicating that acidity promotes tumor progression in prostate cancer [33,34].
Natural remedies for controlling cancer
Warburg hypothesized that unfavorable conditions at the cell level, such as pH imbalances, poor oxygenation, and deficiency in key nutrients, may cause excessive mutations during cellular adaptation to stressful conditions, resulting in abnormal cell functioning. On the contrary, the chances of survival of the affected cells may be significantly higher if there are favorable conditions at the cellular level, in terms of pH level, adequate oxygenation, and availability of good sugars, essential vitamins, minerals, enzymes, and coenzymes that play key roles in regulating cell metabolism, differentiation, and maturation [26].
Particularly, for cancer tumors that have their roots in oxygen deficiency (hypoxia), and/or an acidic cellular environment (acidosis), providing an oxygenated, nutrient-rich environment, with optimum alkalinity at the cellular level, may retain healthy cells and inhibit growth of cancer cells [4,26]. A research study on prostate cancer has indicated that inhibiting external tumor acidity by increasing alkalinity at the cell level may reduce the risk of prostate cancer and help with cancer control [33,34].
Nutrition may be a key factor controlling cellular conditions; hence, dietary habits may have a significant impact on the risk of cancer [35]. A healthy plant-based diet that leads to optimal cellular conditions concerning alkalinity and adequate access to essential nutrients, sufficient oxygenation, as well as a reduced exposure to carcinogens, may lower the tension and stresses at the cell level, leading to the control of cancer cells [4,24,26]. Research studies suggest that living a typical Western lifestyle and consuming unhealthy fast foods and sugar-loaded drinks frequently, as well as a high intake of animal proteins and a low intake of plant-based foods may increase the risk of developing cancer. In contrast, there are shreds of evidence suggesting that traditional healthy diets rich in plant foods can reduce the risk of cancer. Particularly, an appropriate plant-based diet with balanced alkalizing effect can provide essential vitamins, minerals, enzymes, coenzymes, good bacteria, and antioxidants that have protective effects against cancer [24,34].
The risk of cancer may substantially be lowered by considering a healthy plant-based diet that includes adequate consumption of raw almonds, lemons, dates, mangoes, figs, grapes, garlic, red onions, green leafy vegetables, tomatoes, carrots, celery, cucumbers, sprouted wheat, sprouted peas, olive oil, spices and ginger, as a salad, side dish, snack in between meals, or a meal in appropriate combinations with properly cooked healthy vegetarian foods such as legumes, rice, potato, green beans, and green peas. Overall, dietary interventions aimed at reducing consumption of animal proteins, and increasing intake of healthy plant proteins can be beneficial and effective in preventing and controlling cancers [36-38].
Supporting the above findings, are studies that found African-Americans who followed fatty meat-heavy diets (a typical Western diet) had a significantly higher risk of colorectal cancer than those consuming rural African foods rich in beans and vegetables [39], frequently consuming raw garlic can significantly reduce the risk of lung cancer [40], a high intake of animal proteins may be associated with higher risks of various cancer types such as breast, prostate, colorectal, and lung cancers [41], an appropriate plant-base diet significantly reduces the risk of cancer [42], and a high intake of raw and fresh vegetables, fruits, nuts and seeds, has been shown to control cancer in many cases [43].
In addition to following a suitable and healthy diet consisting of natural foods and drinking enough chemical-free water for hydration and detoxification of the body [34], cancer prevention and control also requires dietary restrictions on acidifying foods and beverages such as peanuts, coffee, alcohol, sugary drinks, fried foods, commercial dairy products, processed meats, salt-preserved foods, and pickled vegetables, as well as limiting consumption of packaged processed foods and drinks that contain various chemicals [13].
Knowing the key role of healthy nutrition in cancer prevention, Warburg considered the consumption of natural products and healthy foods as a dietary habit, he prepared his own meals from natural foods, and avoided factory prepared foods and processed foods as much as he could. As a result, despite having a family history of cancer, he successfully avoided cancer throughout his life [20,44].
In conclusions, understanding the origin of cancer and the metabolic pathways in cancer cells can help with metabolic control of the disease.
A fundamental cause of cancer is behavioral changes and mutations in a normal cell under the effect of cellular stress, that leads to a return to the behavior of ancient single-celled organisms, resulting in the cells overconsuming our body’s resources for their own survival and growing out of control.
The risk of developing cancer increases with chronic cellular stress caused by lack of oxygen and key nutrients, as well as exposure to excess acidity and carcinogens.
Providing an oxygen-rich cellular environment with optimal alkalinity, as well as delivering essential nutrients from natural healthy foods to the body cells, may reduce the tension at the cell level, leading to control of the disease, as well as improving body resistance to developing cancers.
A nutrient-rich healthy diet that includes appropriate plant-based foods, along with a healthy life style and reducing exposure to the environmental carcinogens, may be effective in providing appropriate condition at the cell level and reducing the cellular stresses, and help with controlling cell mutations and cancers.
List of abbreviations
ATP: Adenosine triphosphate
Acknowledgements
I would like to thank Dr. Ted Greiner (World Nutrition), Dr. Gordon Edwards (Canadian Coalition for Nuclear Responsibility), Dr. Alexandra Rasnitsyn (University of Toronto), Dr. Ali Karami (National Foundation of Healthy Lifestyle) and Dr. Majid Tafrihi (University of Mazandaran) for sharing valuable information that was used in the study.
This research was performed independently, and did not receive any grant from funding agencies, commercial, or not-for-profit sectors.
Conflict of interest
The author declares that he has no conflicts of interest.
Consent for publication
The manuscript does not contain any individual person’s data.
Ethical Approval
The study was performed using publicly available information in the given references. Hence, ethical approval is not applicable for this study.
References
- The Landscape of the Emergence of Life Jheeta S. Life.2017;7(2). CrossRef
- Origin of Life: The Point of No Return Kunnev D. Life.2020;10. CrossRef
- The Role of Hydrogen Sulfide in Evolution and the Evolution of Hydrogen Sulfide in Metabolism and Signaling Olson KR , Straub KD . Physiology (Bethesda, Md.).2016;31(1). CrossRef
- Understanding the Warburg Effect Yields New Insights into the Metabolic Control of Cancer Bahrami H, Tafrihi M. Journal of Cancer Research Updates.2023;12:44-48. CrossRef
- Glucose Metabolism in Cancer Bose S, Le A. Advances in Experimental Medicine and Biology.2018;1063. CrossRef
- The rise of atmospheric oxygen Kump LR . Nature.2008;451(7176). CrossRef
- (PDF) Understanding Natural Selection: Essential Concepts and Common Misconceptions Gregory TR . ResearchGate.. CrossRef
- Cyanobacteria and the Great Oxidation Event: evidence from genes and fossils Schirrmeister BE , Gugger M, Donoghue PCJ . Palaeontology.2015;58(5). CrossRef
- Complex systems biology Ma'ayan A. Journal of the Royal Society, Interface.2017;14(134). CrossRef
- Understanding Multicellularity: The Functional Organization of the Intercellular Space Bich L, Pradeu T, Moreau J. Frontiers in Physiology.2019;10. CrossRef
- The Cell: A Molecular Approach. Fifth Edition, Publisher: Sinauer Associates, Inc2009. (ISBN-13: 978-0878933006) Cooper MG , Housman RE . 2009.
- Cancer: A Throwback to Ancient Cells? https://torontoriot.com/2019/11/26/cancer-a-throwback-to-ancient-cells/ Rasnitsyn A. 2019.
- Reversing the Warburg effect to control cancer: A review of diet-based solutions Bahrami H, Tafrihi M, Mohamadzadeh S. Journal of Current Oncology and Medical Sciences.2022. https://submission.journalofcoms.com/index.php/JCOMS/article/view/54;2(3).
- Uranium: Known Facts and Hidden Dangers. The World Uranium Hearing conference, Salzburg1992 Edwards G. .
- National Cancer Institute (2021) What Is Cancer? https://www.cancer.gov/about-cancer/understanding/what-is-cancer .
- Cancer Metabolism as a New Real Target in Tumor Therapy Chiaradonna F, Scumaci D. Cells.2021;10(6). CrossRef
- Is cancer a disease set up by cellular stress responses? Aranda-Anzaldo A, Dent MAR . Cell Stress & Chaperones.2021;26(4). CrossRef
- Molecular principles of metastasis: a hallmark of cancer revisited Fares J, Fares MY , Khachfe HH , Salhab HA , Fares Y. Signal Transduction and Targeted Therapy.2020;5(1). CrossRef
- New Clarity on the Warburg Effect. https://www.cancer.gov/research/key-initiatives/ras/ras-central/blog/2021/vander-heiden-warburg-effect Luengo A, Li Z, Heiden MV . 2021.
- Warburg effect(s)-a biographical sketch of Otto Warburg and his impacts on tumor metabolism Otto AM . Cancer & Metabolism.2016;4. CrossRef
- On the origin of cancer cells Warburg O. Science (New York, N.Y.).1956;123(3191). CrossRef
- The Warburg Effect: How Does it Benefit Cancer Cells? Liberti MV , Locasale JW . Trends in Biochemical Sciences.2016;41(3). CrossRef
- “An old idea, revived: Starve Cancer to Death.” https://www.nytimes.com/2016/05/15/magazine/warburg-effect-an-old-idea-revived-starve-cancer-to-death Apple S. The New York Times Magazine.2016.
- (PDF) The Alkaline Diet and the Warburg Effect Bahrami H, Greiner T. ResearchGate.. CrossRef
- Principals of Cancer Genetics Bunz F. Publisher: Springer, Dordrecht.2016. CrossRef
- Improving Natural Resistance to Cancer: An Overview of Metabolic Pathways in Cancer Cells Integrated with Regional Cancer Incidence Statistics Bahrami H, Tafrihi M. Jentashapir Journal of Cellular and Molecular Biology.2023;14(3). CrossRef
- Biology of glucose metabolization in cancer cells Adewale O, Basiru A, Ojo O, Adewale O, Olayide I, Emuowhochere R. Journal of Oncological Sciences.2017;3. CrossRef
- Cancer-generated lactic acid: a regulatory, immunosuppressive metabolite? Choi SYC , Collins CC , Gout PW , Wang Y. The Journal of Pathology.2013;230(4). CrossRef
- Mini-Review: The Influence of Respiratory and pH Imbalance in Cancer Development Drochioiu G. International Journal of Biochemistry Research & Review.2014. CrossRef
- Acidosis Activates Endoplasmic Reticulum Stress Pathways through GPR4 in Human Vascular Endothelial Cells Dong L, Krewson EA , Yang LV . International Journal of Molecular Sciences.2017;18(2). CrossRef
- Acidosis and cancer: from mechanism to neutralization Ibrahim-Hashim A, Estrella V. Cancer Metastasis Reviews.2019;38(1-2). CrossRef
- How and Why Are Cancers Acidic? Carbonic Anhydrase IX and the Homeostatic Control of Tumour Extracellular pH Lee S, Griffiths JR . Cancers.2020;12(6). CrossRef
- Acidity promotes tumour progression by altering macrophage phenotype in prostate cancer El-Kenawi A, Gatenbee C, Robertson-Tessi M, Bravo R, Dhillon J, Balagurunathan Y, Berglund A, et al . British Journal of Cancer.2019;121(7). CrossRef
- Global trends of cancer: The role of diet, lifestyle, and environmental factors Bahrami H, Tafrihi M. Cancer Innovation.2023;2(4). CrossRef
- Metabolic reprogramming in prostate cancer Ahmad F, Cherukuri MK , Choyke PL . British Journal of Cancer.2021;125(9). CrossRef
- Mediterranean diet and health status: Active ingredients and pharmacological mechanisms Schwingshackl L, Morze J, Hoffmann G. British Journal of Pharmacology.2020;177(6). CrossRef
- The Mediterranean Diet as source of bioactive compounds with multi-targeting anti-cancer profile Maruca A, Catalano R, Bagetta D, Mesiti F, Ambrosio FA , Romeo I, Moraca F, et al . European Journal of Medicinal Chemistry.2019;181. CrossRef
- Nutrition for cancer prevention and control: A review of dietary risk factors and protective measures Bahrami H. Journal of Cancer Screening and Prevention.2023;2(4).
- Fat, Fiber and Cancer Risk in African Americans and Rural Africans O'Keefe SJD , Li JV , Lahti L, Ou J, Carbonero F, Mohammed K, Posma JM , et al . Nature communications.2015;6. CrossRef
- Raw garlic consumption as a protective factor for lung cancer, a population-based case-control study in a Chinese population Jin Z, Wu M, Han R, Zhang X, Wang X, Liu A, Zhou J, et al . Cancer Prevention Research (Philadelphia, Pa.).2013;6(7). CrossRef
- Associations between plant-based dietary patterns and risks of type 2 diabetes, cardiovascular disease, cancer, and mortality - a systematic review and meta-analysis Wang Y, Liu B, Han H, Hu Y, Zhu L, Rimm EB , Hu FB , Sun Q. Nutrition Journal.2023;22(1). CrossRef
- Vegetarian diets: what are the advantages? Leitzmann C. Forum of Nutrition.2005;(57). CrossRef
- Raw-eating, A new world free from diseases, vices and poisons. A. Ter Hovannessian, 3rd edition. ASIN: B0007K7W9K Hovannessian AT . 2000.
- The Warburg effect as an adaptation of cancer cells to rapid fluctuations in energy demand Epstein T, Gatenby RA , Brown JS . PloS One.2017;12(9). CrossRef
License

This work is licensed under a Creative Commons Attribution-NonCommercial 4.0 International License.
Copyright
© Asian Pacific Journal of Cancer Biology , 2024
Author Details