Angiogenesis in Breast Cancer: A Review
Download
Abstract
Breast cancer is the leading cause of cancer related deaths worldwide. The development of new blood vessel from preexisting vasculature is associated to the growth and metastasis of breast cancer. The present review aimed to highlight the mechanisms of angiogenesis and the progression of malignancy in breast cancer. Poor breast cancer prognosis correlate with increased micro-vascular density and factors that stimulate vascular growth. Inhibiting angiogenesis of this process reduce the oxygen and nutrient supply. Therefore, tumor angiogenesis is a strategic therapeutic target for preventing breast cancer. We suggest the use of angiogenesis inhibitors to be explored to interrupt the process of tumor development.
Introduction
Breast Cancer is a complex disease that affects millions of women worldwide. It is characterized by the uncontrolled growth and spread of cancerous cells in the breast tissue. According to World Health Organization (WHO), in 2020, about 2.3 million women were diagnosed with breast cancer globally with over 685 000 deaths, and 7.8 million women are at risk, making it the world’s most prevalent cancer among women [1]. Despite several investigations directed toward understanding and controlling breast cancer, it persists as a major health burden both in developed and developing countries [2].
Cancerous cells are often surrounded by stromal cells in the tumor microenvironment. Common stromal cell surrounding the mammary epithelium include fibroblasts, immune cells, and adipocytes. The stromal cell facilitates neoplastic cells to produce pro-angiogenic factors which cause angiogenic activity in blood vessels leading to the formation of new blood vessels to supply oxygen and nutrients to tumor cells [3,4]. The newly formed tumor vasculature is dynamically unstable, hyper permeable, immature with reduced pericyte coverage, and irregular. Angiogenesis is an essential step for the growth and distant metastasis of solid cancers [5]. It has been observed that the release of pro-angiogenic factors such as vascular endothelial growth factor (VEGF), fibroblast growth factor (FGF), angiopoietin, platelet-derived growth factor (PDGF) and the transforming growth factor-β by cancer cells excites the growth of new blood vessels, supplying the tumor with oxygen and nutrients, promoting its survival and metastasis [6]. Inhibiting angiogenesis or targeting existing tumor vessels can be utilized as a treatment approach alongside, as an alternative to conventional chemotherapy. Improved survival of breast cancer is proven in early detection concomitant with effective medical treatments [7]. The present study aimed to highlight the mechanisms of angiogenesis, assessing the factors that trigger angiogenesis, and the progression of malignancy in breast cancer as well as reviewing current knowledge on the inhibitors of angiogenesis as a potential therapeutic agent.
Angiogenesis in Breast Cancer
Angiogenesis encompasses a series of stages by which new blood vessels are formed from existing vessels, allowing the transport of oxygen and nutrients to the body’s tissues [8]. They are established in the earlier stage of vasculogenesis. Angiogenesis continues the growth of the vasculature by processes of sprouting and splitting. It is a requirement for embryonic development and typically occurs during wound healing, but is also necessary for tumor growth and spread in cancer.
Different tumor types, including breast cancer, have proven the vital role of tumor neovascularization. To grow bigger than a few centimeters in diameter, breast cancer like other solid tumors, requires the development of new blood vessels which is the neovascularization [9]. The extra tumor angiogenesis in breast cancer veins not only supplies more nutrients to the tumor, but they also provide possible pathways for tumor distribution and metastasis [10].
Moreover, breast cancer cells, depend on a vascular network of capillaries to provide food and oxygen consistently. Endothelial cells (ECs), which line the internal surface of blood vessels, do not reproduce, hence capillaries do not spread rapidly. Hypoxia which means lack oxygen triggers a variety of transcriptional responses that are facilitated by transcription factors called hypoxia-inducible factors [11]. The hypoxia inducible factors (HIFs) are transcription factors that control the expression of genes involved in physiological processes including angiogenesis, metabolism, and cell division. Local angiogenesis is one of the tumor microenvironment’s long-term major responses to low oxygen levels. It is the fusion of endothelial cell (EC) precursors that leads to the creation of capillary plexus, which thereafter evolves into blood vessels. Angiogenesis is required for a variety of normal processes, including embryonic development, growth, and wound healing [12]. As a result, the tumor activates an angiogenic switch and enters an irreversible active angiogenic state. Because of the tumors’ newly acquired status, they can recruit new capillaries, restoring oxygen and nutrients to both the angiogenic and none angiogenic cells, resulting in rapid tumor growth [13].
Even though surgical excision of tumours is the current standard of care for breast cancer, adjuvant therapy such as anti-angiogenic therapy, has been used after surgery in advanced disease stages when surgery is no longer an option [14]. Angiogenesis-targeting compounds have recently received a lot of attention in breast cancer research. Bevacizumab is a recombinant vascular endothelial growth factor (VEGF) antibody that binds to all known isoforms of VEGF-A and blocks receptor interaction, inhibiting angiogenesis and tumor growth. It was made from a mouse monoclonal antibody that had been humanized [15]. One of the milestones of antiangiogenic treatment, which was first suggested by Judah Folkman many years ago, contributed immensely to this angiogenic factor controlling many of the processes involved in angiogenesis, as well as its importance as a model for the rational design of an anticancer agent. Because all tumors including liquid tumors like leukemia’s are angiogenesis dependent, angiogenesis is highly restricted in adults, the endothelium of the vessels is accessible, and any treatment would be amplified through subsequent tumor necrosis, the antiangiogenic approach has always appealed to researchers. Furthermore, because endothelial cells are non-neoplastic and should have a stable genome, cancer resistance should no longer be an issue [16].
After encouraging results in preclinical trials targeting VEGF, the FDA authorized bevacizumab in 2008 for the treatment of metastatic HER2-negative breast cancer [17]. Following that, multiple anti-angiogenic medicines targeting VEGF or obstructing its receptor’s action were licensed, and they are now routinely utilized in the treatment of various cancers. The FDA, however, cancelled its certification in 2011 due to contradicting results from earlier trials and claims of increased toxicity as a result [18]. While the discovery of these anti-angiogenic drugs ansd small molecules were declared as a success in one aspect of the cancer fight, the agents’ modest activities, such as their inability to arrest recurring tumors in a latent state and the reasonable improvement in overall patient survival, dampened the celebration.
Mechanism of Angiogenesis
Two basic types of angiogenesis exist, sprouting and intussusception [19]. Sprouting angiogenesis represents the major mechanism of angiogenic growth and is characterized by sprouts of endothelial cells growing through the branching morphogenesis process [3,20]. Alternatively, intussusception angiogenesis involves the splitting of existing blood vessels to form new ones [19].
The process of angiogenesis in breast cancer includes the following steps:
Vasodilation: The first step in angiogenesis, is the expansion of the existing blood vessels, which is caused by the release of nitric oxide and other vasodilators.
Extracellular matrix degradation: involves the deterioration of the extracellular matrix (ECM) surrounding the existing blood vessels. This is achieved by the release of proteolytic enzymes such as matrix metalloproteinase (MMPs).
Endothelial cell migration: After the ECM is broken down, endothelial cells (ECs) move towards the site of angiogenesis.
Proliferation: the endothelial cells then reach the site of angiogenesis, they begin to proliferate, and sprout.
Tube formation: Tubes are formed from the sprouts of endothelial cells which eventually become new blood vessels [21].
When stimulating the growth of cells in vitro, angiogenic activators play a major role in starting the process of angiogenesis (Figure 1).
Figure 1. Formation of New Blood Vessels from Pre-existing Vasculature and Proliferation of Endothelial Cells.
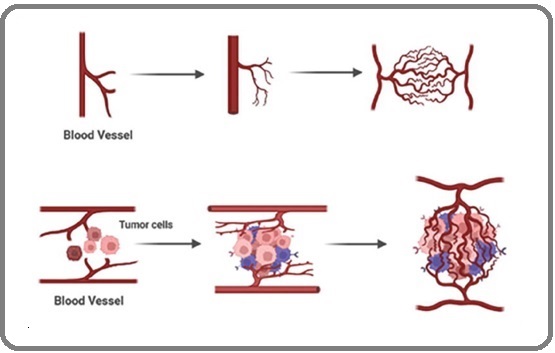
This involves communication between tumor cells and numerous other cell types within the tumor microenvironment [22]. The release of pro-angiogenic growth factors by the tumor cells affects the existing blood vessels, and a balance between pro- and anti-angiogenic factors is maintained in the microenvironment to develop and stabilize the newly formed blood vessels [23].
Tumors can trigger the angiogenic switch by altering the balance between angiogenesis inducers and inhibitors exerting an antagonistic action (Figure 2).
Figure 2. Illustrates the Balance Theory of the Angiogenic Switch.
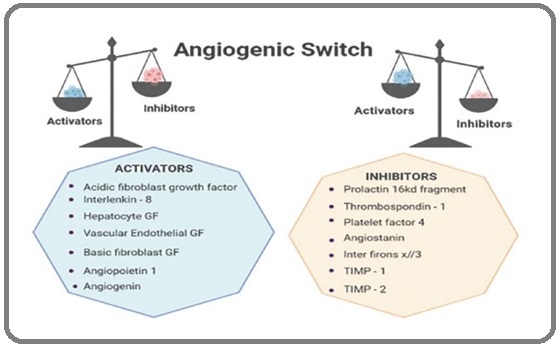
The switch is turned on or off depending on this balance.
The angiogenic switch mechanism of action is considered to be in control of the formation of new blood vessels. In breast cancer, the balance between activators and inhibitors is crucial to maintain the right equilibrium to prevent uncontrolled growth [24]. The growth stimulation of new blood vessels in a tumor is a very complex process that is regulated by hypoxia, that is, reduced oxygen levels at every step [25]. Furthermore, endothelial cells play a crucial role in this process while several studies have continued to show that a protein called HIF-1α along with other members of the HIF family wheels almost every aspect of angiogenesis. In other words, the HIF pathway acts as a master regulator of angiogenesis. For a new blood vessels to emerge, hypoxia and the HIF pathway in the tumor cells are essential. They regulate the expression of various genes that support angiogenesis, including angiopoietin-1, an-giopoietin-2, cytokines, platelet-derived growth factor (PDGF), vascular endothelial growth factor (VEGF), and basic fibroblast growth factor (bFGF). The FGF and vascular endothelial growth factor (VEGF) families are particularly important in the field of neoplastic vascularization as they play a key role in cancer therapy and diagnosis [26].
Vascular Endothelial Growth Factor Pathway
Vascular endothelial growth factor (VEGF-A) was discovered back in 1983 and its complete sequence was determined in 1989. It was the first cytokine identified as a major player to tumor angiogenesis. Initially, it was purified from tumor cell ascites and referred to as vascular permeability factor (VPF) [27]. It was found to have some biological effects on endothelial cell mitogenesis; so it became commonly known as VEGF [28]. Nowadays, VEGF is recognized as a multifunctional peptide that can stimulate the proliferation of endothelial cells and promote angiogenesis both in vivo and in vitro (Figure 3).
Figure 3. The VEGF Signaling Pathway.
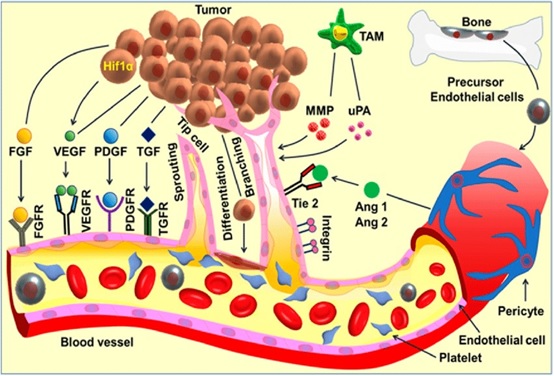
The VEGF family consists of at least five different members, and their effects are mediated through the three VEGF receptors (VEGFR).
Tumor cell causes a complex cascade of angiogenic signaling and activates downstream cellular events in multiple cell types, especially the endothelial cells, leading to angiogenesis. Pro-angiogenic factors include fibroblast growth factor (FGF) families, vascular endothelial growth factor (VEGF), platelet-derived growth factor (PDGF), transforming growth factors-alpha/beta (TGF-α/β), and angiopoietins (Ang. 1 and 2) and the associated receptors. The detachment of the perivascular cells from the mature blood vessels initiates vessel remodeling and endothelial cell proliferation. Platelets become activated and recruited to the sites of the exposed basement membrane. Tumor- associated macrophages (TAM) produce angiogenic factors such as VEGF, MMPs, and urokinase-type plasminogen activator (uPA) whereas, precursor endothelial cells move to the perceived wound site and release angiogenic factors. The activated endothelial cells release proteases that lead to the extracellular matrix (ECM) remodeling followed by directional sprouting. The signaling cascades activate tube formation and branching, followed by vessel arterio- venous patterning and maturation. Cancer progenitor stem cells could differentiate to endothelial cells thereby contributes in angiogenesis [29].
The vascular endothelial growth factor receptors (VEGFR) communicate with the inside of the cells through trans-membrane receptor tyrosine kinases (RTKs) [30]. As soon as VEGF is formed, it binds to its receptor (VEGFR) and ligands on the surface of endothelial cells, this binding activates the trans-membrane tyrosine kinase receptors on the cell surface, which results in dimerization, auto phosphorylation, and activation of the downstream signaling pathway. After this process a series of events take place including;
A. It promotes the survival of endothelial cells
B. It triggers the degradation of basement membrane by proteases;
C. It stimulates the migration of endothelial cells (ECs) into the interstitial space, causing sprouting;
D. Then endothelial cell proliferation at the migrating tip; leading to an increase in endothelial cell proliferation
E. Eventually, lumen formation occurs, generation of new basement membrane with the recruitment of pericytes, the formation of anastomoses, and finally, the establishment of blood flow [31].
EC; endothelial, HIF; hypoxia inducible factor, VEGF; vascular endothelial growth factor.
The process of angiogenesis is strongly regulated by the following factors (Figure 4);
Figure 4. A Schematic Process of Angiogenesis.
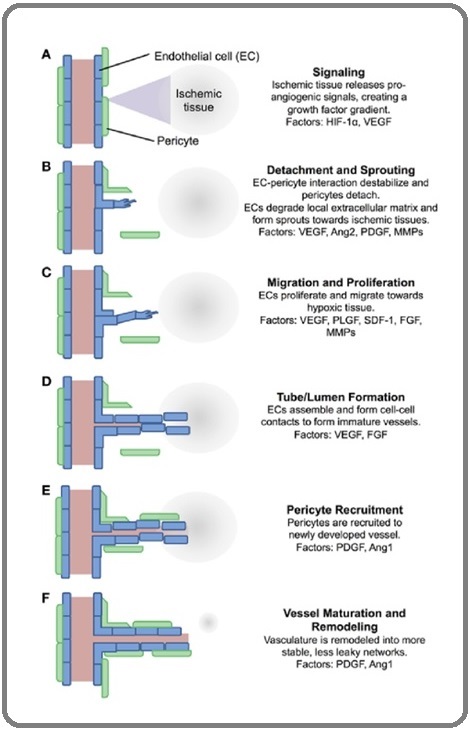
1. Ischemic tissue release proangiogenic signals which diffuse into nearby tissue
2. Pericytes detach from nearby vessels and endothelial cells form sprouts
3. ECs proliferate and migrate towards the signal gradient
4. ECs align into immature vessels
5. Pericytes are recruited to the new vessels
6. Vasculature is remodeled and stabilized
An Increase in the production of pro-angiogenic factors like VEGF and proteolytic enzymes along with a decrease in anti-angiogenic factors leads to the activation of endothelial cells as discussed earlier [32]. In due course, a capillary network is formed, furnishing the growing tumor with the necessary nutrients and oxygen. Through this network, tumor cells could enter the systemic circulation leading to distant metastases. The detection of various angiogenic inducers over the past decade has shed light on the regulatory process of angiogenesis in tumors. Targeting tumor angiogenesis has become an attractive approach for therapy in different types of human tumors which is aimed to inhibit the growth of tumor vessels by interfering with the intracellular signaling of VEGF and VEGFR [33].
Angiogenesis Inhibitors
In breast cancer treatment, inhibiting the growth of blood vessels is been proposed a number of years ago by Judah Folkman as an innovative therapeutic strategy [34]. Studies have explored the use of anti-angiogenic factors and compounds that can decrease the release of pro-angiogenic factors, prevent their binding to receptors, or inhibit their actions. These approaches offer promising anti-angiogenic targets that have been identified include;
1. Inhibition of the growth factors that promote endothelial proliferation
2. Inhibition of the proteases required for endothelial cells to penetrate the basement membrane and form new blood vessels
3. Interruption of specific intracellular signal transduction pathway
4. Initiation of endothelial cell apoptosis or inhibition of EC survival
5. Inhibition of endothelial bone marrow precursor cells
6. Inhibition of αvβ3-integrin-vitronectin interaction that is pivotal in mediating ECs adhesion to ECM during neovascularization[31]. As a result, research has made the inhibition of the VEGF pathway a central focus of angiogenesis therapy [35]. Some of the strategies that have been formulated, other than inhibiting the VEGF pathway include employing antibodies targeting VEGF or VEGFR, use of soluble VEGFR/VEGFR hybrids, and use of inhibitors directed against tyrosine kinase [36].
Natural Inhibitor of Angiogenesis
Neovastat: Neovastat is a substance derived from shark cartilage that blocks the growth of extra blood vessels by competing with VEGF and its receptor. It also inhibits matrix metalloproteinase, activates tissue plasminogen activator enzymatic activities, and triggers cell death in endothelial cells. Neovastat has been found to have significant anti-tumor effects, and experiments have shown that it prevents the formation of blood vessels in implants containing basic fibroblast growth factor (bFGF). Curcumin: which is found in turmeric has been shown anti-angiogenic effects by reducing VEGF production and disrupting signaling pathways [22].
Health Implications of Anti-angiogenic Therapy
It has been reported that angiogenesis inhibitors interfere with several normal body processes such as wound healing [37], blood pressure, kidney function, fetal development, reproduction, and increased the risk of clots formation in the arteries that would result in stroke or heart attack [38]. Some of the effects of anti-angiogenic therapy include the following:
Hypertension: this is one of the most observed side effects of systemic inhibition of VEGF signaling, which is also one of the most manageable side effects with the use of standard anti-hypertensive medications [39]. Treatments of cancer by the inhibition of VEGF signaling will cause endothelial dysfunction by decreasing the level of VEGF which will eventually result in hypertension. Under normal condition, VEGF is known to release vasodilator nitric oxide (NO) in vessel walls by up-regulating endothelial nitric oxide synthase (eNOS) and prostacyclin (PGI2), resulting in vasodilation through the activation of the mitogen-activated protein kinase (MAPK) and phosphatidylinositol 3-kinase (PI3K) downstream pathways [40]. Therefore, by inhibiting VEGF, the production of NO will be decreased which will promote vasoconstriction, increase peripheral resistance, and eventually raise the blood pressure [41].
Because angiogenesis is required for wound healing, high levels of VEGF are produced during the repair of normal wounds. It has been reported that the inhibition of VEGF in angiogenesis therapy could interfere with normal angiogenesis and cause a disruption in the angiogenic process of wound healing. In this regard, the treatment of patients having metastatic colorectal cancer with bevacizumab showed an increase in post-surgical wound healing complications, including wound dehiscence and impaired wound healing [42]. It was thought earlier that anti-angiogenesis strategies by blocking tumor angiogenesis would limit the permeability of its own and other adjunct therapies. However, data demonstrated that treatment with anti-angiogenic drugs results in a more efficient normalized vasculature that might allow for improved tumor delivery of drugs [43].
Angiogenic Markers
Understanding and assessing angiogenesis in breast cancer has different methods. It can be quantified by invasive and noninvasive methods.
Analysis of angiogenic markers: This assesses the diagnostic performance of angiogenesis markers in tumors and their reflecting levels in the serum of breast cancer patients. Angiogenin, Ang.2, fibroblast growth factor basic, intercellular adhesion molecule (ICAM)-1, keratinocyte growth factor (KGF), platelet-derived growth factor-BB, and VEGF- A are measured using a growth factor multiplex protein assay.
Immunohistochemistry: Immunohistochemistry is a technique where the corresponding antibodies are used to detect specific proteins in tissue samples. It helps to identify the expression of angiogenesis-related proteins in breast cancer tissue samples by classifying several molecular subtypes [44].
Gene expression profile: Used to diagnose angiogenesis in breast cancer. It categorizes breast carcinomas into the molecular subtypes with significant variations in occurrence, risk factors, prognosis, and treatment reactivity.
Biopsy and Histopathology: A biopsy is performed to collect tissue specimens for measurement of quantitative parameters by light or electron microscopy of blood vessels and histological examination of tissue sections stained with endothelial antibody or blood flow with intravascular markers such as colloidal carbon, India ink, and high molecular weight trackers for the presence of blood vessels. This is classified as an invasive method [45]. Noninvasive methods include: Magnetic resonance imaging, functional computed tomography, and positron emission tomography scanning. Noninvasive methods in comparison with invasive methods have a much lower resolution and generally cannot expose the vessels of the micro circulation. Many non-invasive techniques are developed for humans [17].
In conclusion, angiogenesis occurring within tumors plays a crucial role in breast cancer development and metastasis. Targeting this critical process shows a promising therapeutic method for breast cancer. However, the effectiveness of angiogenesis inhibitors could be at variance across the different tumor types. Consequently, more research is required to better understand the mechanisms and improved the effectiveness of antiangiogenic therapies. In addition, it is important to identify the biomarkers that could predict response to treatment for personalized approaches and reducing the menace of breast cancer.
Abbreviations
WHO, World Health Organization; VEGF, Vascular Endothelial Growth Factor; FGF, Fibroblast Growth Factor; PDGF, angiopoietin Platelet-Derived Growth Factor; VEGF-A, Vascular endothelial growth factor; ICAM, Intercellular adhesion molecule; KGF, Keratinocyte growth factor; NO, Nitric oxide; eNOS, endothelial nitric oxide synthase; PG, Prostacyclin; MAPK, Mitogen-activated protein kinase; PI, Phosphatidylinositol; PRISMA ScR, Preferred Reporting Items for Systematic Reviews and Meta Analyses extension for Scoping Review guidelines.
Acknowledgments
Statement of Transparency and Principals:
· Author declares no conflict of interest
· Study was approved by Research Ethic Committee of author affiliated Institute.
· Study’s data is available upon a reasonable request.
· All authors have contributed to implementation of this research.
References
- Angiogenesis and Breast Cancer. Cancers (Basel) Sergiusz Łukasiewicz , Adhemar Longatto Filho , Jose ́ Manuel Lopes , Fernando C . 2021.
- The changing global patterns of female breast cancer incidence and mortality Bray F, McCarron P, Parkin DM . Breast Cancer Research.2004;6(6). CrossRef
- Tumor Angiogenesis and Anti-Angiogenic Strategies for Cancer Treatment Teleanu RI , Chircov C, Grumezescu AM , Teleanu DM . Journal of Clinical Medicine.2019;9(1). CrossRef
- Vascular Endothelial Cell Biology: An Update Krüger-Genge A, Blocki A, Franke R, Jung F. International Journal of Molecular Sciences.2019;20(18). CrossRef
- Targeting pericytes for angiogenic therapies Kelly-Goss MR , Sweat RS , Stapor PC , Peirce SM , Murfee WL . Microcirculation (New York, N.Y.: 1994).2014;21(4). CrossRef
- Carmeliet and Jain. Angiogenesis in Breast Cancer: Mechanisms, Clinical Significance, and Therapeutic Perspectives” (2011), published in Cold Spring Harbor Perspectives in Biology .
- Night blindness and associated factors among pregnant women in Ethiopia: A systematic review and meta-analysis | Ethiopian Medical Journal W/tsadik DS , Lerango TL , Bekele BB , et al . .
- Siamakpour-Reihani, in Translational Advances in Gynecologic Cancers, 2017. A.A. Secord, S . .
- Recent translational research: Antiangiogenic therapy for breast cancer: Where do we stand? Miller KD . Breast Cancer Research.2004;6:128-132.
- Angiogenesis Folkman J, Shing Y. The Journal of Biological Chemistry.1992;267(16).
- Breast cancer statistics, 2013 DeSantis C, Ma J, Bryan L, Jemal A. CA: a cancer journal for clinicians.2014;64(1). CrossRef
- Angiogenic targets for potential disorders Bhadada SV , Goyal BR , Patel MM . Fundamental & Clinical Pharmacology.2011;25(1). CrossRef
- Angiogenesis in life, disease and medicine Carmeliet P. Nature.2005;438(7070). CrossRef
- Patterns and emerging mechanisms of the angiogenic switch during tumorigenesis Hanahan D, Folkman J. Cell.1996;86(3). CrossRef
- RIBBON-1: randomized, double-blind, placebo-controlled, phase III trial of chemotherapy with or without bevacizumab for first-line treatment of human epidermal growth factor receptor 2-negative, locally recurrent or metastatic breast cancer Robert NJ , Diéras V, Glaspy J, Brufsky AM , Bondarenko I, Lipatov ON , et al . Journal of Clinical Oncology: Official Journal of the American Society of Clinical Oncology.2011;29(10). CrossRef
- A cancer therapy resistant to resistance Kerbel RS . Nature.1997;390(6658). CrossRef
- Targeting angiogenesis: progress with anti-VEGF treatment with large molecules Grothey A, Galanis E. Nature Reviews. Clinical Oncology.2009;6(9). CrossRef
- Anti-angiogenesis in cancer therapy: Hercules and hydra Bellou S, Pentheroudakis G, Murphy C, Fotsis T. Cancer Letters.2013;338(2). CrossRef
- Angiogenesis. San Rafael (CA): Morgan & Claypool Life Sciences; 2010. PMID: 21452444. Adair TH , Montani J. .
- Tumor angiogenesis: causes, consequences, challenges and opportunities Lugano R, Ramachandran M, Dimberg A. Cellular and Molecular Life Sciences.2020;77(9). CrossRef
- Angiogenesis: basic pathophysiology and implications for disease Felmeden DC , Blann AD , Lip GYH . European Heart Journal.2003;24(7). CrossRef
- Curcumin attenuates angiogenesis in breast cancer through VEGF inhibition Johnson C, Thompson D, et al . Molecular Cancer Research.2019;10(2):21.
- Intricacies of bevacizumab-induced toxicities and their management Gressett SM , Shah SR . The Annals of Pharmacotherapy.2009;43(3). CrossRef
- Tumour Angiogenesis in Breast Cancer Singh P, Basalingappa M, K Gopenath TS , Sushma BV . IntechOpen.2022. CrossRef
- Pericytes at the intersection between tissue regeneration and pathology Birbrair A, Zhang T, Wang Z, Messi ML , Mintz A, Delbono O. Clinical science (London, England : 1979).2015;128(2). CrossRef
- Angiogenic signaling pathways and anti-angiogenic therapy for cancer Liu Z, Chen H, Zheng L, Sun L, Shi L. Signal Transduction and Targeted Therapy.2023;8(1). CrossRef
- Vascular endothelial growth factor: much more than an angiogenesis factor Senger DR . Molecular Biology of the Cell.2010;21(3). CrossRef
- The biology of VEGF and its receptors Ferrara N, Gerber H, LeCouter J. Nature Medicine.2003;9(6). CrossRef
- Recent Advancements of Nanomedicine towards Antiangiogenic Therapy in Cancer Mukherjee A, Madamsetty VS , Paul MK , Mukherjee S. International Journal of Molecular Sciences.2020;21(2). CrossRef
- Expression of vascular endothelial growth factor (VEGF) family members in breast cancer Kurebayashi J, Otsuki T, Kunisue H, Mikami Y, Tanaka K, Yamamoto S, Sonoo H. Japanese Journal of Cancer Research: Gann.1999;90(9). CrossRef
- Mechanism and its regulation of tumor-induced angiogenesis Gupta MK , Qin R. World Journal of Gastroenterology.2003;9(6). CrossRef
- The role of angiogenesis in solid tumours: An overview Makrilia N, Lappa T, Xyla V, Nikolaidis I, Syrigos K. European journal of internal medicine.2009;20. CrossRef
- Breast cancer-induced angiogenesis: multiple mechanisms and the role of the microenvironment Boudreau N, Myers C. Breast Cancer Research.2003;5(3).
- Targeting Angiogenesis in Breast Cancer: Current Evidence and Future Perspectives of Novel Anti-Angiogenic Approaches Ayoub NM , Jaradat SK , Al-Shami KM , Alkhalifa AE . Frontiers in Pharmacology.2022;13. CrossRef
- Vascular Cells in Blood Vessel Wall Development and Disease Mazurek R, Dave JM , Chandran RR , Misra A, Sheikh AQ , Greif DM . Advances in Pharmacology (San Diego, Calif.).2017;78. CrossRef
- Fibroblast Growth Factor Signaling in the Vasculature Yang X, Liaw L, Prudovsky I, Brooks PC , Vary C, Oxburgh L, Friesel R. Current atherosclerosis reports.2015;17(6). CrossRef
- Adverse effects of anticancer agents that target the VEGF pathway Chen HX , Cleck JN . Nature Reviews. Clinical Oncology.2009;6(8). CrossRef
- The Role of Angiogenesis in Cancer Treatment Rajabi M, Mousa SA . Biomedicines.2017;5(2). CrossRef
- Management of hypertension in angiogenesis inhibitor-treated patients Izzedine H, Ederhy S, Goldwasser F, Soria JC , Milano G, Cohen A, Khayat D, Spano JP . Annals of Oncology: Official Journal of the European Society for Medical Oncology.2009;20(5). CrossRef
- Possible molecular mechanisms involved in the toxicity of angiogenesis inhibition Verheul HMW , Pinedo HM . Nature Reviews. Cancer.2007;7(6). CrossRef
- Mechanisms of angiogenesis Karamysheva AF . Biochemistry. Biokhimiia.2008;73(7). CrossRef
- Gerritsen, in Microcirculation, 2008 25. KA. Thomas, in Encyclopedia of Cell Biology, 2016 Mary E. .
- Dual-action combination therapy enhances angiogenesis while reducing tumor growth and spread Wong P, Demircioglu F, Ghazaly E, Alrawashdeh W, Stratford MRL , Scudamore CL , Cereser B, et al . Cancer Cell.2015;27(1). CrossRef
- Mechanisms of Tumor Angiogenesis. In: Marmé, D. (eds) Tumor Angiogenesis Zuazo-Gaztelu I , Casanovas O . Springer, Cham.2019. CrossRef
- A Review on Angiogenesis and Its Assays Tahergorabi Z, Khazaei M. Iranian Journal of Basic Medical Sciences.2012;15(6).
License

This work is licensed under a Creative Commons Attribution-NonCommercial 4.0 International License.
Copyright
© Asian Pacific Journal of Cancer Biology , 2024
Author Details