Biosynthesis and Characterization of Silver Nanoparticles Using Cleistanthus collinus; Investigation on Molecular Mechanism of Apoptosis Cell Death Against Lung Cancer Cells
Download
Abstract
Background: The discovery of novel molecular and cellular signaling pathways for cancer medicines would enhance the efficacy of cancer therapy. In the current study, we made an effort to explore the mitochondrial-mediated apoptosis cell death signaling pathway in A549 lung cancer cells by silver nanoparticles (AgNPs) synthesized from leaf extract of Cleistanthus collinus.
Methods: In-depth, A549 lung cancer cells were treated with AgNPs, and further studies such as HOECHST 33342, AO/EB, Rhodamine-123 staining, flowcytometry, RT-PCR, and Western blotting techniques (24, 48, and 72 h) evidenced apoptosis pathway in cancer cells.
Results: Indeed, the microscopic studies proved that AgNPs-treated lung cancer cells appeared with cell shrinkage, membrane swelling, and apoptotic body formation whereas the untreated cells (control) failed to show any morphological consequences. The flow cytometry analysis revealed the G2/M phase of cell cycle arrest by cells were accumulated in large numbers. The RT-PCR data confirmed the expression (Bax and p53) and suppression (Bcl 2) apoptosis-responsive genes in cancer cells. Finally, immunoblotting proved the increased expression of cytochrome c, initiator caspase 9, and executioner caspase 3, which are effective proteins of intrinsic apoptosis activation.
Conclusion: Ultimately, the overall present investigations confirmed the mitochondrial signaling pathway played a key role in inducing apoptosis in A549 cancer cell death and demonstrated that AgNPs are novel therapeutic agents for cancer nanomedicine
Introduction
In the past few decades, lung cancer is the most common causes of morbidity and mortality in both sexes worldwide among the other cancers types [1]. The outcome of therapeutic efficiency of lung malignancy is still lacking and present chemotherapeutic methods are unsuccessful to increase the survival rate of cancer patients because of decreased bioavailability, need high-dose, harmful complications, poor therapeutic indices, arising of multiple drug resistance, and address the non-specific targeting [2]. Hence, its essential to discover a novel clinical approaches for lung cancer treatment which can be achieved by molecular-targeted therapies. The green nanotechnology is an emerging field which shown to bridge the obstacle of biological and physical sciences by employing various nanomaterials at dynamic fields of science. The nanobiotechnology has proven the possibility of targeted drug delivery at specific cells using biologically synthesized nanoparticles [3]. In recent times, the nanomedicines have been investigated for the targeted drug delivery to cure large number of diseases, particularly cancers [4].
In the past decade, the programmed cell death, especially apoptosis pathway is one of the crucial mechanism for cancer therapy. Generally, apoptosis showed a spectacular role in removal of unwanted cells to conserve the healthy balance between cell survival and death in metazoan [5, 6]. Indeed, understanding the molecular mechanisms of apoptotic pathway is a very essential process for therapeutic potential against various threatening diseases. In cancer condition, there is a failure of equilibrium between cell division and cell death by fault in acquire the signaling is leading cause of tumor progression and result to resistance of tumors for anticancer drug molecules [7]. In recent time, a number of researches have concluded that down regulated expression of tumour suppressor gene p53 which consequences in weakened apoptotic cell death and enhanced tumour growth and development [8] and also proven, inactivation of p53 in normal cells, connected to many human cancers progression [9, 10]. Moreover, the induction of mitochondrial singnals playing a crucial function to activate apoptosis by enhance the level of caspase proteins and other effector molecules [11, 12].
The ability of chemotherapeutic agents to induce apoptosis in tumor cells has become a therapeutic approach which may be lead to side effects, since, the development of novel approaches for cancer treatment is essential [13]. Generally, various research studies suggested that metabolites derived from plants may possess pro-apoptotic properties and have great potential for possible applications in cancer prevention [14, 15]. Indeed, plenty of literature studied on anticancer activity of AgNPs [16-18] although most of them are failed to assess molecular mechanisms of cell death by quantify the expression of genes and proteins involved in programmed cell death pathway. Hence, the present investigation was designed to explore the cellular and molecular mechanisms of biologically synthesized AgNPs in induction of mitochondrial mediated apoptosis pathway in human lung cancer cells.
Materials and Methods
Collection and Preparation of plant Extract
The healthy leaves of Cleistanthus collinus (C. collinus) was collected at Kolli Hills, Namakkal District, Tamil Nadu, India. The leaves were unsoiled and cut into small pieces followed by cleaned with distilled water and shade dried for three weeks. After drying, samples were made into fine powder and sealed into plastic bags until further use.
Synthesis and characterization of AgNPs
For the synthesis of AgNPs nanoparticles, 1 mM silver nitrate (HIMEDIA) solution was mixed with aqueous leave extract and initially colour change was determined. Further, the characterizations of AgNPs were done using Synergy HT Multi-Mode Microplate Reader (Bio-Tek Instruments, Inc., Winooski, VT, USA), which recorded between the wavelength range of 300 to 700 nm. Functional groups were examined using Fourier transform infrared spectroscopy by JASCO (FT/IR-6200) spectrophotometer, operating within a wavelength range of 400 to 4000 cm−1. TEM and dynamic light scattering technique with Zetasizer Nano-ZS (Malvern instruments Ltd., Malvern, UK). The other characteristic datas of AgNPs were previously reported by Kanipandian et al. [19] and summarized in Table 1.
Techniques used | Results |
UV-visible spectroscopy | 420 nm |
FT-IR for functional groups analysis | Revealed the major peaks which are confirmed the presence of N–H aromatic secondary amine and attributed to the N–H stretching, methyl C–H asym./sym. stretching, methane C–H stretching and aldehyde, also C–O primary alcohol as well as C–O stretch and disulfides (C–S stretch) linkage of proteins respectively |
XRD | Face centered cubic structure (JCPDS File No: JCPDS File No. 03-0921) and crystalline nature with 22 nm size |
EDX | Showed a dominant peak at ~3 eV was associated with the silver. Also, observed that the elemental compositions of silver (78.17%) and chlorine (21.83%) |
FESEM and HRTEM | Spherical shape with size ranging from 20 to 40 nm. |
SAED | Confirm the crystalline nature of the particles and similar with XRD pattern |
A549 cell line culture
The A549 lung cancer cells were cultured in Dulbecco’s Modified Eagles Medium (DMEM) with supplemented with 2 mM L-glutamine and 10% fetal bovine serum (GIBCO, USA), Balanced Salt Solution (BSS) adjusted to contain 1.5 g/l Na2CO3, 0.1 mM non-essential amino acids, 1 mM sodium pyruvate, 2 mM L-glutamine, 1.5 g/l glucose, 10 mM HEPES. Penicillin and streptomycin (100 IU/100 µg) were added to the medium and adjusted to 1 ml/l. Proliferative cultures were incubated at 37°C in a humidified 5% CO2 incubator and subculture carried out by washing the cell monolayers twice with calcium and magnesium-free phosphate buffered saline (PBS) followed by addition of 1x Trypsin/EDTA solution and incubation at 37° C until the cells detached.
Cell viability analysis
MTT assay (3- (4,5 - dimethylthiazolyl-2)-2,5- diphenyltetrazolium bromide) was performed to identify the cytotoxicity effect of AgNPs against A549 cells. IC50 concentration is about 30 µg/ml and it is reported earlier by Kanipandian et al., [19]. Hence, this study was further extended by using 30 µg/mL concentration to detect the molecular mechanisms of AgNPs induced apoptotic cancer cell death pathway.
HOECHST 33342 staining
The cell death by apoptosis was examined by staining with Hoechst 33342 and fluorescence microscopy analysis [20]. The A549 cells were seeded in 6 well plates and treated with biogenic AgNPs with 30 µg/mL (IC50) for different time intervals (24, 48 and 72 h). At each time point, the medium was replaced and the cells were cleaned twice with phosphate buffered saline (PBS), fixed in 4% para-formaldehyde for 20 min. Then, the cells were re-washed, and stained with HOECHST 33342 (10 µg/ml) at 37 ºC for 20 min in the dark. Then stains were washed with methanol followed by PBS, and the cells were subjected to detect any nuclear morphological alterations and apoptotic bodies under blue channel fluorescence with fluorescent microscopy (Nikon Eclipse, Inc., Japan).
Analysis for apoptosis induction
Alterations in cell morphology treated with AgNPs was identified using acridine orange and ethidium bromide (AO/EB) fluorescence staining [21]. Concisely, the dye mixture containing 1 µl of (100 mg/ml of AO and 100 mg/ ml of EB in distilled water) was directly stained with cells grown on clean microscope cover slips. After staining, the cells were washed with PBS (pH 7.2) and incubated for 1 min, the cells were visualized immediately under a fluorescence microscope (Nikon Eclipse, Inc., Japan) at 400× magnification with an excitation filter at 480 nm.
Mitochondrial membrane stability (∆ψm)
The mitochondrial membrane potential (∆ψm) was identified by staining with rhodamine 123 which is a cationic fluorophore taken up by mitochondria as a 50 result of their membrane potential. The changes in ∆ψm as a result of mitochondrial depolarization were measured by modified method [22]. The A549 cells were seeded in 6 well plates (1×105 cells/well) and exposed to IC concentration of green synthesized AgNPs. After the specific time intervals (24, 48 and 72 h), the cells were fixed in 4% paraformaldehyde, washed twice with PBS, and added specific stain Rh-123 (10 µg/ml) for 30 min at 37 ºC. The cells were then washed twice with methanol to remove the excess stain, washed again with PBS, and analyzed for alterations in ∆ψm using fluorescence microscope with an excitation and emission wavelengths (505 nm and 534 nm).
Flow cytometry
Here, we attempted to perform flow cytometry analysis to distinguish AgNPs treated cells in different phases of the cell cycle [23]. The A549 cancer cells (1×106) were seeded in each tissue culture dish and allowed to grow for overnight. The cells were treated with AgNPs for 72 h and harvested by trypsinization then centrifuged at 2500 rpm for 5 min at RT (room temperature). The cells in pellet were re-suspended in 300 µL of PBS–EDTA to which 700 µl of cold 70% was added drop wise to the pellet while vortexing. This should ensure fixation of all cells and minimize clumping, and the samples were stored at 4 ºC for overnight. Subsequently, 1:100 volumes of 20 mg/ml RNase were added, it will confirm only DNA, not RNA, is stained and the mixture was incubated at 37 ºC for 1h. Propidium iodide was added to a final concentration of 50 µg/ml and incubated for 10–20 min at room temperature. Finally, the stained cells were analyzed for DNA histograms and for cell cycle phase distribution using flow cytometry (Becton Dickinson Immuno cytometry System, USA).
Semi-quantitative RT- PCR
The alterations in the expression pattern of apoptosis-related genes such as Bax, Bcl-2, p53 and β-actin genes were analyzed using RT-PCR technique [11]. Here, TriZol reagent was used to extract the total RNA from AgNPs treated A549 cells. Briefly, the cDNA was synthesized using a cDNA synthesis kit and amplified according to the manufacturers protocols in a 25 µl reaction mixture containing random primer pairs (1.0 µl): 10× buffer (5.0 µl), cDNA (2.0 µg), 25 mM/l MgCl (3.0 µl), 10 mM/l dNTPs (1.0 µl), and Taq polymerase (2.5 U). Semi-quantitative RT-PCR amplification cycles possess denaturation at 94 ºC for 1 min, primer annealing at 57 ºC for 45 s and extension at 72 ºC for 45 s, for a total of 30 cycles followed by final extension at 72 ºC for 10 min. The amplified products were analyzed on 1.5% agarose gel electrophoresis and densitometric analysis of bands was done by ImageJ Software. The details of primer sequences used in this study are given in Table 2.
Genes | Primer sequences | Size (bp) |
Bax | Forwrd-5´-TTTGCTTCAGGGTTTCATCC-3´ | 246 |
Reverse-5´-CAGTTGAAGTTGCCGTCAGA-3´ | ||
Bcl-2 | Forward-5´-CATCCATTATAAGCTGTCGCA-3´ | 498 |
Reverse-5´-TGCCGGTTCAGGTACTCAGT-3´ | ||
p53 | Forward-5´-GCTCTGAC TGTACCACCATC-3´ | 619 |
Reverse-5´-CTTCTGACGCACACCTATTG-3´ | ||
β-Actin | Forward-5´- GTTGCTATCCAGGCTGTGC-3´ | 540 |
Reverse-5´- GCATCCTGTCGGCAATGC-3´ |
Immunoblotting analysis
The expression of apoptotic proteins such as cytochrome c, caspases 9 and 3 in A549 cells was examined by Western blotting technique [24]. The cells (2×106) were seeded onto 100-mm culture dishes in the presence or absence of AgNPs. After 20 min, the lysates were homogenized using cytosol extraction buffer, then centrifuged at 12,000 rpm for 10 min at 4 ºC. The Proteins were estimated (30 µg/lane) by Bradford method and lysates were mixed with 4X loading dye containing 5% β-mercaptoethanol followed by boiling at 95°C for 5 min and subjected to 10% SDS-PAGE separation and then electrotransferred onto PVDF membrane. Subsequently, the membranes were blocked in TBST solution containing 5% (w/v) non-fat milk for 2h, followed by overnight incubation with primary antibodies such as caspase 9 and 3, cytochrome c and β-actin at 4 ºC. Next day, blots were washed with TBST and incubated with secondary antibodies conjugated with horse radish peroxidase for 1 h at room temperature. The antigen-antibody complex was detected using an ECL chemiluminescence kit. Quantification of specific protein signals were performed using ImageJ software and were normalized to the signal intensity of β-actin.
Statistical analysis
All the experiments were carried out in triplicates and the data were expressed as mean ± SEM. The difference between means was analyzed by one-way ANOVA. All statistical analyses were performed using SPSS 17.0 software. A level of p ≤ 0.05, p ≤ 0.01was taken as statistically significant.
Results
The characteristic results of TEM analysis confirmed the spherical structure which is showed in Figure 1 (a & b).
Figure 1. Depicts the Colour Change of Reaction Mixture and TEM (a and b), size (c) and Zeta Potential (d) Characterization of Silver Nanoparticles .
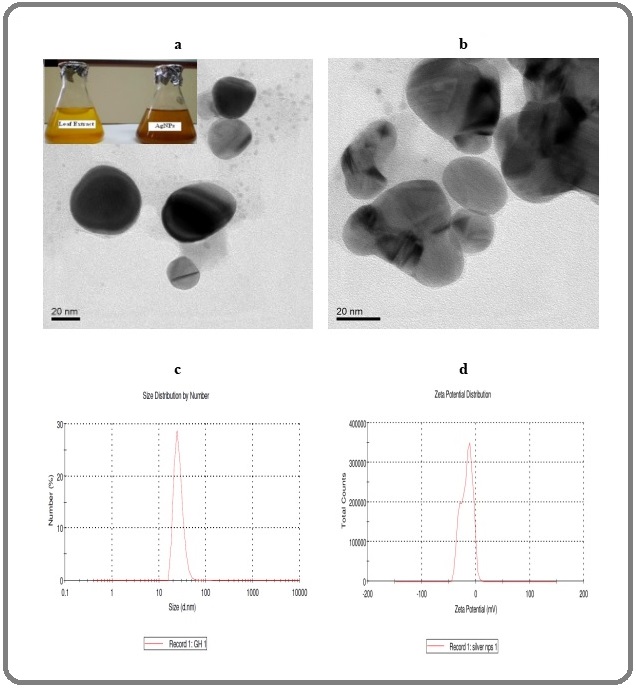
The mean size of the AgNPs was between 20 and 40 nm measured by DLS. The zeta potential measurement demonstrated the surface charges of AgNPs and indicated a value of -21 mV (Figure 1c and d) and it shows about surface charge and stability of the particles. In this study, we have evaluated the anticancer potential of AgNPs against human lung cancer cells (A549) and normal cells (HBL-100) in vitro to reveal the mechanism of cell death pathway.
HOECHST 33342 staining
Cell death induced by AgNPs was further analyzed with the help HOECHST 33342 which is of fluorescent DNA binding dye. HOECHST 33342 is known to form fluorescent complexes with natural double-stranded DNA and is helpful to find out the apoptotic nuclei. Surprisingly, staining with HOECHST 33342 clearly shown the induction of apoptosis upon treatment of cancer cells with AgNPs whereas, control cells (untreated) showed normal cell morphology. Further, apoptotic nuclei of treated cells were identified by reduced nuclear size, condensed chromatin gathering at the periphery of the nuclear membrane and a total fragmented morphology of nuclear bodies with bright blue color emission (Figure 2).
Figure 2. Study on Apoptosis Induction in A549 Lung Cancer Cells at IC50 Concentration (30 µg/ml) for 24, 48 and 72 h. HOECHST 33342 staining shows the nuclear fragmentation.
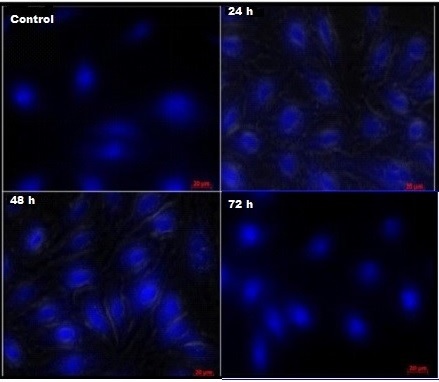
AO/EtBr fluorescence staining
The morphological alterations on AgNPs exposed cells were observed using AO/EtBr fluorescence staining (Figure 3).
Figure 3. Demonstrate the AO/EtBr Stained Respective Control Cells Appear in Live Cells with Green Color and Orange Colored Apoptotic Cells and Necrotic Cells Appearing in Red Color.
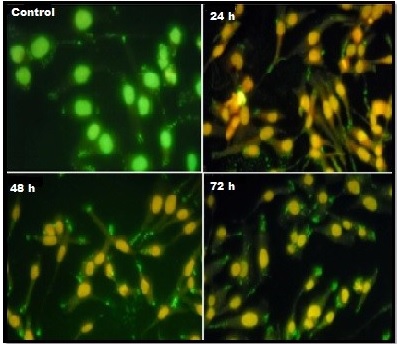
Interestingly, the results of this study clearly demonstrated that number of viable cells decreased when increasing the treatment duration. The AO/EtBr study explored the apoptotic cells comprising the condensed form of nuclei and apoptotic bodies were stained orange whereas the necrotic cells were stained red and the untreated cells were stained uniform green color. Amazingly, the number of cells stained red did not increase since, clearly indicated that most of the cells were not undergoing necrosis and cell death occurred primarily through apoptosis.
Rhodamine 123 staining
The loss of membrane potential (∆ψm) was determined by decline in mean fluorescence intensity level following the treatment of A549 cells with AgNPs for various time lengths (24, 48 and 72 h), then visualized under fluorescence microscope. Interestingly, the cells shown a time-dependent decrease in Rh-123 fluorescent intensity was observed. The fluorescence study evidenced the loss of ∆ψm, the images are given in the Figure 4.
Figure 4. Rhodamine-123 Staining Clearly Indicates the Loss of Mitochondrial Membrane Potential. The panel of images indicates decrease in fluorescence intensity with increasing the treatment duration confirmed the loss of mitochondrial membrane stability which facilitates the release of cytochrome c..
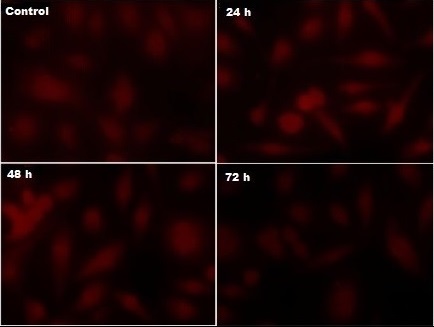
Cell cycle arrest
The effect of AgNPs on cell cycle processes was analyzed using flow cytometry with propidium iodide (PI) staining. The characteristic histograms for the cell cycle distribution following exposure to AgNPs are shown in Figure 5.
Figure 5. (a) Cell Cycle Alteration in A549 Cancer Cells after Treatment with AgNPs ans Analyzed by Flow Cytometry. This examination indicates that cell cycle arrest in the G2/M phase (b) Densitometry analysis associated with an increased abundance of cell distributions. The results are represented as the mean ± SD of three independent experiments (p ≤ 0.05).
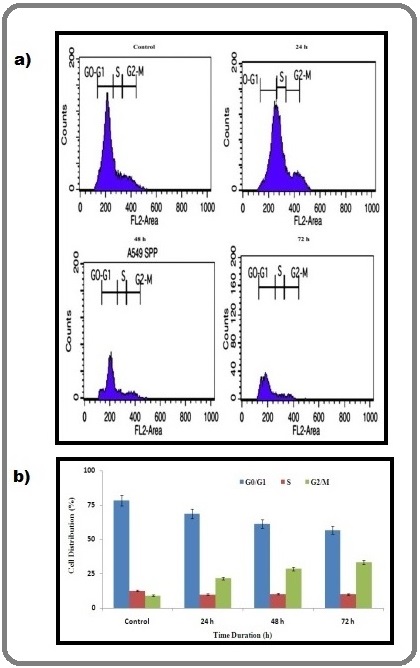
The treatment of A549 cells with AgNPs for 72 h resulted in a decrease of the G0/G1 phase compared with the control. The percentage of S phase in treated cells enhanced relative to that of control cells. Interestingly, the flow cytometry analysis also revealed the effect of AgNPs on the aggregation of cells in the G2/M phases accompanied by cell cycle arrest.
Effect of AgNPs on apoptotic gene expression
Here, the investigation made to detect the important mechanism underlying gene expression profile of both anti-apoptotic and apoptotic using semi quantitative RT-PCR. The A549 cells were allowed to expose into AgNPs for various time durations (24, 48 and 72 h). At ending of each time point, total apoptotic (Bax and p53) and anti-apoptotic genes (Bcl-2) were isolated, and expression levels were measured. Amazingly, RT-PCR study revealed a significant reduction in the expression of bcl-2 and an upregulation in the expression of bax and p53 in cancer cells treated with AgNPs when compared to untreated control cells. Interestingly, we ascertained that the expression of apoptotic genes are time dependent, which shown increasing the expression pattern with increased time of treatment with AgNPs (Figure 6).
Figure 6. (a) Semi-quantitative RT-PCR Analysis of Intrinsic Apoptotic Related Gene Expressions of Cells. The treated cells were harvested and analyzed for apoptotic and anti-apoptotic gene expression. (b) Densitometry analysis of intrinsic apoptotic related gene expressions of cells treated with AgNPs for 24 h, 48 h and 72 h. β-Actin gene was used as an internal loading control. The results shown here are representative of three independent experiments .
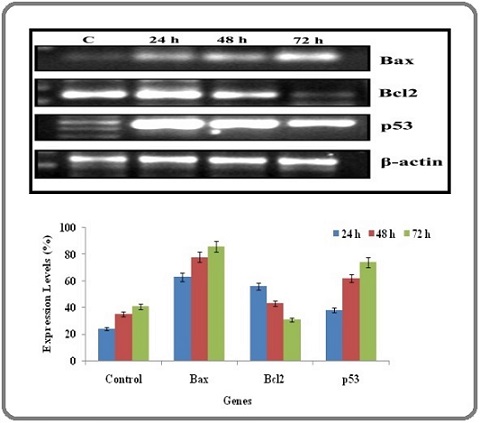
Furthermore, the down regulated expression of bcl-2 confirmed that AgNPs has the biomedical potential to trigger the intrinsic apoptotic cancer cell death by inhibiting the expression of anti-apoptotic genes. In this study, we used β-actin as an internal control and it does not displayed any alterations in the expression pattern.
Induction of Caspases by AgNPs
Immunoblotting analysis was carried out to detect whether the apoptotic target gene expression is reflected at the protein level in the AgNPs treated cancer cells. Here, we evaluated the apoptotic protein expression level of cytochrome c, caspase -9, caspase -3 and β-actin used as internal control for this study. Interestingly, the blotting patteren shown the up-regulated expression of apoptotic sensitive proteins like cytochrome c, caspase -9, caspase -3 were significantly increased with time dependent manner, depicted in Figure 7.
Figure 7. (a) AgNPs Induced Mitochondrial Mediated Apoptosis, Wherein the Protein Expression Profile was Investigated by Immnoblotting. It clearly indicates that mitochondrial protein such as caspase 9, caspase 3 and cytochrome c expression was increased and β-Actin was used as an internal control. (b) Densitometry showed apoptotic protein expression for AgNPs treated cells after 24 h, 48 h and 72 h. The results showed here are representative of three independent experiments.
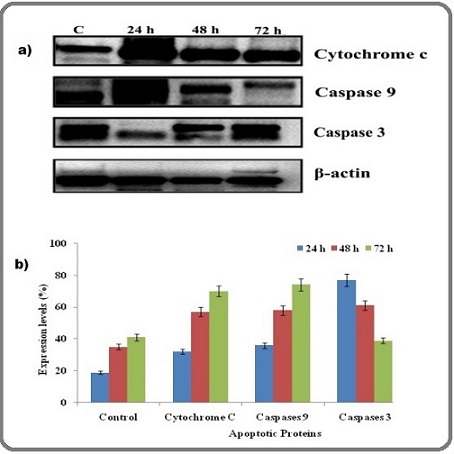
Densitometry analysis proven that the percentage of protein expression level was increased when increasing the treatment duration of A549 lung cancer cells to AgNPs compare with untreated cells. Excitedly, the present report found that up-regulated expression of intrinsic apoptotic proteins such as cytochrome c, caspase -9, caspase -3 with time dependent manner.
Discussion
The Hoechst 33342 staining clearly indicated cell grwoth arrest by distinct nuclear physiological changes in the AgNPs treated A549 cancer cells. The formation of apoptosis was defined by several morphological hallmarks of apoptosis in the nucleus including chromatin condensation, nuclear fragmentation which are accompanied by membrane blebbing, DNA fragmentation and cleavage of key cellular proteins [25]. Interestingly, the results of this study showed that AgNPs suppressed the cancer cell growth via morphological alterations. The similar result was reported in HCT-116 colon cancer cell line exposed to gold nanoparticles [26]. Apoptosis is reasoned to be the primary cell death mechanism which takes place in consequence to cytotoxicity of the cells [6]. The present investigation made clear evidence of anti-proliferation effect of AgNPs would be associated with their potential to activate apoptosis signals facilitates cell death in the cancer. The AO/EtBr fluorescence staining clearly evidenced morphological changes on AgNPs exposed lung cells. This examination is concurrent with many earlier reports proven that late apoptotic or necrotic cells have broken membrane that facilitate the invading of ethidium bromide to intercalate into DNA and emit fluoresce red [27].
The loss of membrane potential (∆ ψ m) in the silver nanoparticles treated A549 cells observed by decrease in mean fluorescence intensity level. The mechanisms for AgNPs induced toxicity may be related with mitochondrial damage, oxidative stress, DNA damage and induction of apoptosis [28]. The current observation distinctly indicated that the activation of apoptosis in cancer cells by AgNPs was accompanied by modification in the mitochondrial membrane potential. The results provided the clear clue that AgNPs interrupted the normal functions of mitochondria at the early stages of apoptosis, subsequently co-ordinate caspase 3 stimulation through the cleavage of caspases by the release of cytochrome c which caused the cell death. The optimal quantity of mitochondrial membrane potential is an basal element for cell survival; the loss of ∆ ψ m is a distinguishing feature of the early phase of apoptosis [29]. The failure of mitochondrial membrane stability followed by depolarization and dysfunctions would leads to cancer cell death [22]. The present work revealed that fluorescence intensity was weakened with increasing the time interval of the treatment with AgNPs (time dependent). The corresponding effects were discovered in human hepatocarcinoma cells (HepG2) exposed to CuO NPs which showed that decreased fluorescent intensity indicates the significant reduction of mitochondrial membrane potential [30]. Another study elucidated gold nanoparticles significantly increased the level of ROS, in effect causing mitochondrial membrane sensitization to trigger the cascade of apoptosis in breast cancer cells [31]. The silver nanoparticles has a potential to increase the level of reactive oxygen species and hydroxyl radical may attributes to mitochondrial dysfunctional and indication of antifungal effects through apoptosis [32]. This experimental finding implied that the AgNPs induced apoptosis in A549 cells via G2/M phase cell cycle arrest in time-dependent. A few recent publication suggested that AgNPs caused cancer cell apoptosis, cell cycle arrest and decreased viability of colon cancer cells in vitro [33, 34].
RT-PCR data revealed that there was a significant reduction in the expression of Bcl-2 and an upregulation in the expression of bax and p53 in AgNPs treated A549 cells. The Bcl-2 family included pro-apoptotic and anti-apoptotic that play a key role in the regulation of apoptosis [35] especially via the intrinsic pathway which act mainly at the mitochondria level [36-38]. Besides, the present study found that up-regulated expression of p53 which increased dramatically in time dependent manner in the AgNPs treated cancer cells. Hence, the current results also demonstrated that AgNPs induced apoptosis in A549 cells through activation of p53 and mitochondria-mediated apoptosis pathway. The p53 tumor suppressor gene has fundamental role participating in cell cycle regulation, DNA repair, and programmed cell death. Since, these gene expression studies coinciding with many existing literature suggest that p53 activated signals leads to caspase activation [39-41].
Interestingly, the present study found that up-regulated expression of intrinsic apoptotic proteins such as cytochrome c, caspase -9, caspase -3 with time dependent manner. The current outcome concur with a previous study of McBride et al. [42] examined that mitochondria are involved in major roles such as signaling, cellular differentiation, cell death, as well as the control of the cell cycle and cell growth. Likewise, stated that interruption and regulation of permeability of mitochondrial outer membrane leads to the activation of cascade of caspases by the release of some apoptotic stimuli [43,44]. The caspase 3 is reasoned as an execution protein for apoptotic cell death pathway and hence we assessed the expression profile and it showed up-regulated in the biogenic AgNPs treated A549 cells. The overall our investigation suggest that up-regulation of cytochrome c might triggered the expression of initiator caspase i.e. caspases 9 and executioner caspase called caspase 3 into their cleaved form by causing loss of mitochondrail membrane stability. Finally, the cleaved caspase 3 execute apoptosis cell death pathway followed by DNA damage and nuclear condensation. The previous research suggested that mitochondria might activate the intrinsic apoptotic signaling pathway in cancer cells by alterations in the protein expression patterns [45]. To date, there are 11 or 12 caspases are identified in humans, among them caspase 3 plays a key enzyme in the execution-phase of cell apoptosis [46, 47]. The mitochondria-mediated pathway facilitates by the release of cytochrome c led to the loss of mitochondrial membrane potential, formation of apoptosome, resulting in characteristic morphologic changes in the nucleus, finally activation of caspase 3 and fragmentation of DNA [11, 48, 49].
In conclusion, we demonstrated the biological potential of AgNPs from Cleistanthus collinus for anticancer activity in A549 lung cancer cells. From this commencement investigation, we conclude that AgNPs effectively acted as a nanodrug to activate mitochondrial signaling mediated cell death apoptotic pathway. Here, we found that intrinsic apoptotic pathway was associated with chromatin condensation, nuclear fragmentation, membrane blebbing and DNA fragmentation leads to cell death. Also examined that loss of membrane stability causes releasing of cytochrome c into cytosol from mitochondrial inner membrane space leads down expression of bcl-2, up-regulation of bax and finally induction of caspases are the key mechanisms to induce cell death in cancer cells. This current report gives a comprehensive research on AgNPs mediated apoptosis cell death in human lung cancer cells and its mechanisms, on AgNPs attributed to induce intrinsic apoptotic pathway for targeting lung carcinogenesis. From, this overall findings, we conclude that AgNPs can be used as a vehicle of targeted treatment against many human cancers.
Ethics approval and consent to participate
“Not applicable” in this section
Consent for publication
“Not applicable” in this section
Availability of data and materials
“Not applicable”
Funding
“Not applicable” in this section
Authors contributions
Nagarajan Kanipandian: conceptualization, writing - review and editing the manuscript. Ramar Ramesh: review and editing the manuscript.
Acknowledgements
“Not applicable” in this section
Conflict of interest
The author declare that there is no conflict of interest to disclose.
References
- World Health Organization. 2020. https://www.who.int/news-room/fact-sheets/ detail/cancer Accessed 31 January 2022.
- Controlled drug delivery vehicles for cancer treatment and their performance Senapati S, Mahanta AK , Kumar S, Maiti P. Signal Transduction and Targeted Therapy.2018;3. CrossRef
- HER2 Targeted Breast Cancer Therapy with Switchable “Off/On” Multifunctional “Smart” Magnetic Polymer Core–Shell Nanocomposites, Vivek, R, Thangam, R, Rajesh kumar, S, Rejeeth, C, Senthil Kumar, G, Sivasubramanian, S, Vincent, S, Gopi, D, Kannan S. Appl Mat Int.2016;8:2262-67.
- Nanotechnology in cancer diagnosis: progress, challenges and opportunities Zhang Y, Li M, Gao X, Chen Y, Liu T. Journal of Hematology & Oncology.2019;12(1). CrossRef
- Apoptotic cell death in disease-Current understanding of the NCCD 2023 Vitale I, Pietrocola F, Guilbaud E, Aaronson SA , Abrams JM , Adam D, Agostini M, Agostinis P, et al , Bazan Nicolas G., Becker Christoph, Bernassola Francesca, Bertrand Mathieu J. M., Bianchi Marco E., Blagosklonny Mikhail V., Blander J. Magarian, Blandino Giovanni, Blomgren Klas, Borner Christoph, Bortner Carl D., Bove Pierluigi, Boya Patricia, Brenner Catherine, Broz Petr, Brunner Thomas, Damgaard Rune Busk, Calin George A., Campanella Michelangelo, Candi Eleonora, Carbone Michele, Carmona-Gutierrez Didac, Cecconi Francesco, Chan Francis K.-M., Chen Guo-Qiang, Chen Quan, Chen Youhai H., Cheng Emily H., Chipuk Jerry E., Cidlowski John A., Ciechanover Aaron, Ciliberto Gennaro, Conrad Marcus, Cubillos-Ruiz Juan R., Czabotar Peter E., D'Angiolella Vincenzo, Daugaard Mads, Dawson Ted M., Dawson Valina L., De Maria Ruggero, De Strooper Bart, Debatin Klaus-Michael, Deberardinis Ralph J., Degterev Alexei, Del Sal Giannino, Deshmukh Mohanish, Di Virgilio Francesco, Diederich Marc, Dixon Scott J., Dynlacht Brian D., El-Deiry Wafik S., Elrod John W., Engeland Kurt, Fimia Gian Maria, Galassi Claudia, Ganini Carlo, Garcia-Saez Ana J., Garg Abhishek D., Garrido Carmen, Gavathiotis Evripidis, Gerlic Motti, Ghosh Sourav, Green Douglas R., Greene Lloyd A., Gronemeyer Hinrich, Häcker Georg, Hajnóczky György, Hardwick J. Marie, Haupt Ygal, He Sudan, Heery David M., Hengartner Michael O., Hetz Claudio, Hildeman David A., Ichijo Hidenori, Inoue Satoshi, Jäättelä Marja, Janic Ana, Joseph Bertrand, Jost Philipp J., Kanneganti Thirumala-Devi, Karin Michael, Kashkar Hamid, Kaufmann Thomas, Kelly Gemma L., Kepp Oliver, Kimchi Adi, Kitsis Richard N., Klionsky Daniel J., Kluck Ruth, Krysko Dmitri V., Kulms Dagmar, Kumar Sharad, Lavandero Sergio, Lavrik Inna N., Lemasters John J., Liccardi Gianmaria, Linkermann Andreas, Lipton Stuart A., Lockshin Richard A., López-Otín Carlos, Luedde Tom, MacFarlane Marion, Madeo Frank, Malorni Walter, Manic Gwenola, Mantovani Roberto, Marchi Saverio, Marine Jean-Christophe, Martin Seamus J., Martinou Jean-Claude, Mastroberardino Pier G., Medema Jan Paul, Mehlen Patrick, Meier Pascal, Melino Gerry, Melino Sonia, Miao Edward A., Moll Ute M., Muñoz-Pinedo Cristina, Murphy Daniel J., Niklison-Chirou Maria Victoria, Novelli Flavia, Núñez Gabriel, Oberst Andrew, Ofengeim Dimitry, Opferman Joseph T., Oren Moshe, Pagano Michele, Panaretakis Theocharis, Pasparakis Manolis, Penninger Josef M., Pentimalli Francesca, Pereira David M., Pervaiz Shazib, Peter Marcus E., Pinton Paolo, Porta Giovanni, Prehn Jochen H. M., Puthalakath Hamsa, Rabinovich Gabriel A., Rajalingam Krishnaraj, Ravichandran Kodi S., Rehm Markus, Ricci Jean-Ehrland, Rizzuto Rosario, Robinson Nirmal, Rodrigues Cecilia M. P., Rotblat Barak, Rothlin Carla V., Rubinsztein David C., Rudel Thomas, Rufini Alessandro, Ryan Kevin M., Sarosiek Kristopher A., Sawa Akira, Sayan Emre, Schroder Kate, Scorrano Luca, Sesti Federico, Shao Feng, Shi Yufang, Sica Giuseppe S., Silke John, Simon Hans-Uwe, Sistigu Antonella, Stephanou Anastasis, Stockwell Brent R., Strapazzon Flavie, Strasser Andreas, Sun Liming, Sun Erwei, Sun Qiang, Szabadkai Gyorgy, Tait Stephen W. G., Tang Daolin, Tavernarakis Nektarios, Troy Carol M., Turk Boris, Urbano Nicoletta, Vandenabeele Peter, Vanden Berghe Tom, Vander Heiden Matthew G., Vanderluit Jacqueline L., Verkhratsky Alexei, Villunger Andreas, Karstedt Silvia, Voss Anne K., Vousden Karen H., Vucic Domagoj, Vuri Daniela, Wagner Erwin F., Walczak Henning, Wallach David, Wang Ruoning, Wang Ying, Weber Achim, Wood Will, Yamazaki Takahiro, Yang Huang-Tian, Zakeri Zahra, Zawacka-Pankau Joanna E., Zhang Lin, Zhang Haibing, Zhivotovsky Boris, Zhou Wenzhao, Piacentini Mauro, Kroemer Guido, Galluzzi Lorenzo**************. Cell Death and Differentiation.2023;30(5). CrossRef
- Apoptosis and Molecular Targeting Therapy in Cancer Hassan M, Hidemichi W, Ali AA , Yusuke O, Noriaki S. BioMed Research Inter.2014;1:1-23.
- Mutant p53 drives metastasis and overcomes growth arrest/senescence in pancreatic cancer Morton JP , Timpson P, Karim SA , Ridgway RA , Athineos D, Doyle B, Jamieson NB , et al . Proceedings of the National Academy of Sciences of the United States of America.2010;107(1). CrossRef
- How does p53 induce apoptosis and how does this relate to p53-mediated tumour suppression? Aubrey BJ , Kelly GL , Janic A, Herold MJ , Strasser A. Cell Death and Differentiation.2018;25(1). CrossRef
- The p53 pathway in breast cancer Gasco M, Shami S, Crook T. Breast cancer research: BCR.2002;4(2). CrossRef
- p53 mutations in colorectal cancer Rodrigues N. R., Rowan A., Smith M. E., Kerr I. B., Bodmer W. F., Gannon J. V., Lane D. P.. Proceedings of the National Academy of Sciences of the United States of America.1990;87(19). CrossRef
- Activation of intrinsic apoptotic signaling pathway in cancer cells by Cymbopogon citratus polysaccharide fractions Thangam R, Sathuvan M, Poongodi A, Suresh V, Pazhanichamy K, Sivasubramanian S, Kanipandian N, et al . Carbohydrate Polymers.2014;107. CrossRef
- Apoptosis pathways in cancer and cancer therapy Debatin K. Cancer immunology, immunotherapy: CII.2004;53(3). CrossRef
- Apoptosis as a measure of chemosensitivity to cisplatin and taxol therapy in ovarian cancer cell lines Gibb R. K., Taylor D. D., Wan T., O'Connor D. M., Doering D. L., Gerçel-Taylor C null. Gynecologic Oncology.1997;65(1). CrossRef
- Cytotoxic and apoptotic activity of Cheilanthes farinosa (Forsk.) Kaulf. against human hepatoma, Hep3B cells Radhika NK , Sreejith P. S., Asha V. V.. Journal of Ethnopharmacology.2010;128(1). CrossRef
- Chemopreventive and anti-cancer properties of the aqueous extract of flowers of Butea monosperma Choedon T, Shukla SK , Kumar V. Journal of Ethnopharmacology.2010;129(2). CrossRef
- A feasible approach to phyto-mediated synthesis of silver nanoparticles using industrial crop Gossypium hirsutum (cotton) extract as stabilizing agent and assessment of its in vitro biomedical potential Kanipandian N, Thirumurugan R. Industrial Crops and Products.2014;55. CrossRef
- Biogenic synthesis and spectroscopic characterization of silver nanoparticles using leaf extract of Indoneesiella echioides: in vitro assessment on antioxidant, antimicrobial and cytotoxicity potential Kuppurangan G, Karuppasamy B, Nagarajan K, Krishnasamy Sekar R, Viswaprakash N, Ramasamy T. Applied Nanoscience.2016;6. CrossRef
- An evidence on G2/M arrest, DNA damage and caspase mediated apoptotic effect of biosynthesized gold nanoparticles on human cervical carcinoma cells (HeLa) Murugaraj J, Renganathan A, Gnanasekar S, MubarakAli D, Rajesh M., Sivanandhan G, Kapildev G, et al . Materials Research Bulletin.2014;52. CrossRef
- Characterization, antioxidant and cytotoxicity evaluation of green synthesized silver nanoparticles using Cleistanthus collinus extract as surface modifier Kanipandian N, Kannan S, Ramesh R, Subramanian P, Ramasamy T. Materials Research Bulletin.2014;49. CrossRef
- Alkaloids extracted from Pterogyne nitens induce apoptosis in malignant breast cell line Duarte RA , Mello ER , Araki C, Bolzani VDS , Siqueira e Silva DH , Regasini LO , Silva TGA , et al . Tumour Biology: The Journal of the International Society for Oncodevelopmental Biology and Medicine.2010;31(5). CrossRef
- Mechanistic insight into the rapid one-step facile biofabrication of antibacterial silver nanoparticles from bacterial release and their biogenicity and concentration-dependent in vitro cytotoxicity to colon cells Verma SK , Jha E, Sahoo B, Panda P, Thirumurugan A, Parashar S, Suar M. RSC Advances.2017;7. CrossRef
- Capsaicin production by Alternaria alternata, an endophytic fungus from Capsicum annum; LC-ESI-MS/MS analysis Devari S, Jaglan S, Kumar M, Deshidi R, Guru S, Bhushan S, Kushwaha M, et al . Phytochemistry.2014;98. CrossRef
- Glucose capped silver nanoparticles induce cell cycle arrest in HeLa cells Panzarini E, Mariano S, Vergallo C, Carata E, Fimia GM , Mura F, Rossi M, et al . Toxicology in vitro: an international journal published in association with BIBRA.2017;41. CrossRef
- An investigation on the cytotoxicity and caspase-mediated apoptotic effect of biologically synthesized silver nanoparticles using Podophyllum hexandrum on human cervical carcinoma cells Jeyaraj M, Rajesh M, Arun R, MubarakAli D, Sathishkumar G, Sivanandhan G, Dev GK , et al . Colloids and Surfaces. B, Biointerfaces.2013;102. CrossRef
- Classification of cell death: recommendations of the Nomenclature Committee on Cell Death Kroemer G., El-Deiry W. S., Golstein P., Peter M. E., Vaux D., Vandenabeele P., Zhivotovsky B., et al . Cell Death and Differentiation.2005;12 Suppl 2. CrossRef
- Gold nanoparticles induce apoptosis in HCT-116 colon cancer cell line Maddah A, Ziamajidi N, Khosravi H, Danesh H, Abbasalipourkabir R. Molecular Biology Reports.2022;49(8). CrossRef
- Apoptosis and cell cycle arrest of human colorectal cancer cell line HT-29 induced by vanillin Ho K, Yazan LS , Ismail N, Ismail M. Cancer Epidemiology.2009;33(2). CrossRef
- Cytotoxic effect of Green synthesized silver nanoparticles using Melia azedarach against in vitro HeLa cell lines and lymphoma mice model Sukirtha R, Priyanka KM , Antony JJ , Kamalakkannan S, Thangam R, Gunasekaran P, Krishnan M, Achiraman S. Process Biochemistry.2012;47(2). CrossRef
- Silver Nanoparticles Potentiates Cytotoxicity and Apoptotic Potential of Camptothecin in Human Cervical Cancer Cells Yuan Y, Zhang S, Hwang J, Kong I. Oxidative Medicine and Cellular Longevity.2018;2018. CrossRef
- Copper oxide nanoparticles induced mitochondria mediated apoptosis in human hepatocarcinoma cells Siddiqui MA , Alhadlaq HA , Ahmad J, Al-Khedhairy AA , Musarrat J, Ahamed M. PloS One.2013;8(8). CrossRef
- Gold nanoparticles induced apoptosis via oxidative stress and mitochondrial dysfunctions in MCF-7 breast cancer cells Kowsalya E, Mosachristas K, Jaquline RI, Balashanmugam P, Thiyagarajan D. Applied Organometallic Chemistry.2020;35. CrossRef
- Silver nanoparticles induce apoptotic cell death in Candida albicans through the increase of hydroxyl radicals Hwang I, Lee J, Hwang JH , Kim K, Lee D. The FEBS journal.2012;279(7). CrossRef
- Cytotoxicity of biologically synthesized silver nanoparticles in MDA-MB-231 human breast cancer cells Gurunathan S, Han JW , Eppakayala V, Jeyaraj M, Kim J. BioMed Research International.2013;2013. CrossRef
- Synthesis and characterization of silver nanoparticles using crystal compound of sodium para-hydroxybenzoate tetrahydrate isolated from Vitex negundo. L leaves and its apoptotic effect on human colon cancer cell lines Durai P, Chinnasamy A, Gajendran B, Ramar M, Pappu S, Kasivelu G, Thirunavukkarasu A. European Journal of Medicinal Chemistry.2014;84. CrossRef
- Apoptosis: A review of pro‐apoptotic and anti‐apoptotic pathways and dysregulation in disease O'Brien MA , Kirby R. Journal of Veterinary Emergency and Critical Care.2008;18(6). CrossRef
- Cloning of the chromosome breakpoint of neoplastic B cells with the t(14;18) chromosome translocation Tsujimoto Y., Finger L. R., Yunis J., Nowell P. C., Croce C. M.. Science (New York, N.Y.).1984;226(4678). CrossRef
- Bcl-2 family proteins: regulators of apoptosis and chemoresistance in hematologic malignancies Reed J. C.. Seminars in Hematology.1997;34(4 Suppl 5).
- BCL-2 family members and the mitochondria in apoptosis Gross A., McDonnell J. M., Korsmeyer S. J.. Genes & Development.1999;13(15). CrossRef
- p53 is required for radiation-induced apoptosis in mouse thymocytes Lowe S. W., Schmitt E. M., Smith S. W., Osborne B. A., Jacks T.. Nature.1993;362(6423). CrossRef
- p53 induces apoptosis by caspase activation through mitochondrial cytochrome c release Schuler M., Bossy-Wetzel E., Goldstein J. C., Fitzgerald P., Green D. R.. The Journal of Biological Chemistry.2000;275(10). CrossRef
- Apoptotic efcacy of multifaceted biosynthesized silver nanoparticles on human adenocarcinoma cells Blassan PGA , K Neeraj, K, A Heidi, A, Suprakas SR . Scientific Reports.2018;8(1). CrossRef
- Mitochondria: more than just a powerhouse McBride HM , Neuspiel M, Wasiak S. Current biology: CB.2006;16(14). CrossRef
- Green tea component, catechin, induces apoptosis of human malignant B cells via production of reactive oxygen species Nakazato T, Ito K, Ikeda Y, Kizaki M. Clinical Cancer Research: An Official Journal of the American Association for Cancer Research.2005;11(16). CrossRef
- The BCL-2 protein family: opposing activities that mediate cell death Youle RJ , Strasser A. Nature Reviews. Molecular Cell Biology.2008;9(1). CrossRef
- Multiple pathways of cytochrome c release from mitochondria in apoptosis Gogvadze V, Orrenius S, Zhivotovsky B. Biochimica Et Biophysica Acta.2006;1757(5-6). CrossRef
- Differential modulation of endotoxin responsiveness by human caspase-12 polymorphisms Saleh M, Vaillancourt JP , Graham RK , Huyck M, Srinivasula SM , Alnemri ES , Steinberg MH , et al . Nature.2004;429(6987). CrossRef
- Apoptosis and cancer: mutations within caspase genes Ghavami S., Hashemi M., Ande S. R., Yeganeh B., Xiao W., Eshraghi M., Bus C. J., et al . Journal of Medical Genetics.2009;46(8). CrossRef
- Effect of silver nanoparticles in the induction of apoptosis on human hepatocellular carcinoma (HepG2) cell line Ahmadian E, Dizaj SM , Rahimpour E, Hasanzadeh A, Eftekhari A, Hosain Zadegan H, Halajzadeh J, Ahmadian H. Materials Science & Engineering. C, Materials for Biological Applications.2018;93. CrossRef
- Induction of intrinsic apoptotic signaling pathway in A549 lung cancer cells using silver nanoparticles from Gossypium hirsutum and evaluation of in vivo toxicity Kanipandian N, Li D, Kannan S. Biotechnology Reports (Amsterdam, Netherlands).2019;23. CrossRef
License

This work is licensed under a Creative Commons Attribution-NonCommercial 4.0 International License.
Copyright
© Asian Pacific Journal of Cancer Biology , 2024
Author Details