Plant Secondary Metabolites Inhibit Cancer by Targeting Epidermal Growth Factor Receptor (EGFR): An Updated Review on their Regulation and Mechanisms of Action
Download
Abstract
Cancer is an exceedingly pervasive disease currently, with approximately 14 million individuals diagnosed every year. The lifestyle and environmental changes are the most widespread causes of cancer. There are numerous cancer treatments available, including chemotherapy, radiotherapy, and hormone therapies. However, these procedures have adverse effects. In such circumstances, plant-based therapies have shown increased efficacy. Plant secondary metabolites such as alkaloids, polyphenols, cannabinoids, and flavonoids have anti-inflammatory, anti-tumor, and anti-proliferative properties, making them ideal candidates for cancer treatment. They inhibit major signaling pathways like MAPK, EGFR, VEGF, Ras/Raf, NF-kβ, induce necrosis, apoptosis, ferroptosis, and cause cell cycle arrest. Phytochemicals together with nanomedicines have a better possibility of eliminating malignant cells. They impede mitophagy while regulating Caspase-dependent cascades. Molecular investigation has revealed that they influence DNA repair, liposomal activities, and the phagocytosis process. The highlights of this review encompass how chemotherapeutic agents induce multidrug resistance, and phytochemical-based cancer treatments and their mechanisms of action, including how they rejuvenate cell damage and eliminate tumor cells from the body.
Introduction
Cancer is commonly characterized as the uncontrolled growth or proliferation of cells. Various variables, such as alterations in lifestyles, environmental influences, and genetic predisposition, might contribute to the development of cancer in the population. Immune dysfunction is a significant contributing element to the progression of cancer. An individual with compromised immune function is more vulnerable and susceptible to developing cancer during the span of their lives [1].
1.1 Pervasiveness of cancer
Cancer is an important factor contributing to morbidity and mortality worldwide, leading to a substantial disease burden [2]. Global Cancer Statistics (GCS) 2020 indicate that breast cancer had the highest incidence, with 2.3 million new cases, representing 11.7% of all cancers. GCS 2020 show that breast cancer accounts for 11.7% of all cancers, followed by lung cancer (11.4%), colorectal cancer (CRC) (10.0%), and lung cancer (1.8 million deaths, 18%), followed by CRC (9.4%) and liver cancer (8.3%) [2]. Cancer has been a major issue in Pakistan, provoking serious concerns. The World Health Organization reports that cancer incidence in Pakistan is steadily increasing [3].
1.2 Benefits of plant-based therapies
Numerous plant secondary metabolites, including tannins, flavonoids, and triterpenoids, have been demonstrated to block angiogenesis in cancer cells [4]. Cancer experts have thus concentrated their efforts on natural products as an alternate means of combating cancer and treatment. They exhibit diverse anti-inflammatory and antioxidant properties [5]. Antioxidant properties encompass the cessation of free radical chains and the chelation of redox-active metal ions that induce lipid peroxidation. These attributes also contribute to cancer prevention. Bioactive substances demonstrate anticancer effects either autonomously or synergistically with other agents by modulating metabolic and signalling pathways, inhibiting enzymes essential for cancer progression, angiogenesis, microtubule assembly, and causing apoptosis [6].
1.3 Limitations of plant-based therapies
Since the onset of the pandemic, numerous research have been conducted to identify plant extracts and phytochemicals that are beneficial in managing viral infections, sometimes in conjunction with traditional medications. A primary issue in utilizing natural chemicals for disease treatment is their limited dissolution and bioavailability, confounding clinical research. Encapsulation or conjugation of plant-based secondary metabolites with nanocarriers can effectively enhance medication delivery, biological distribution, biodegradability, and bioavailability [7].
1.4 Types of cancers
There are two types of cancer: benign and malignant tumors. In the event of a benign tumor, the tumor (cluster of cells) endures in one location and doesn’t influence other organs. While malignant tumors have the capacity to invade healthy tissues and organs, they can have serious repercussions. The position of tumor cells, their growth, and the shape and dimension of the tumor are the essential factors in determining whether it is a cancer cell and what type of cancer cells it is. The most prevalent types of cancer increasing across the world encompass lung, breast, pancreatic, skin, prostate, neck, oral, colorectal, and brain tumors [8]. Including all of these sorts, having an offspring later in life renders women more predisposed to cancer owing to various body changes occur as a result of the aging process. Gestational malignancies include cervix, melanoma, breast, thyroid, and lymphoma [9].
1.5 How cancers cell progress and proliferate
Cancer cells advance through a variety of signaling channels. These pathways may differ across different types of tumors. In the general pathway, reactive oxygen species and their levels play a significant role in tumor cell proliferation and development, as well as triggering the carcinogenesis process. Tumor cells adapt to high sulfur metabolic activity by following pentose phosphate pathways, resulting in enhanced NADPH generation. This oxidative stress would lead to the activation of MAPK pathways, among others, for tumor progression [10].
Treatment catastrophes unfold due to the microenvironment surrounding the tumor or cancer cells. The microenvironment expresses the non-cancerous cells present in the tumor and how they respond when a treatment is targeted to them. It also comprises immune cells and blood vessel cells. Drugs can be targeted using fluorescent drug delivery approaches, and various types of cancer cells can be identified [11]. Scientists are striving to develop sustainable immune treatments and medications to inhibit cancer cell development by better understanding the origins and potential of this fatal disease. Nanoparticles are crucial as medication delivery vehicles for this purpose since other biochemical agents impact tumor cells in addition to causing off-target effects. Along with their permeation as well as retention activities, their photolytic capabilities have shown incredible potential for cancer treatments.
1.6 Role of nanoparticles and nanovesicles in cancer treatment
Gold nanoparticles are gaining traction due to their non-immunogenic and harmless impact on the body. Multifunctional and very stable gold nanoparticles have resilient permeability and retention activity, acting directly on the target areas. They are utilized in both pre and post-diagnostic clinical settings to cure the ailments [12]. Recently, microscopic vesicles termed exosomes, which are released by the cell itself, have been probed as an immunological treatment for cancer patients. These nanovesicles play a role in each tumor regulation and inhibition. They have both an anticancer role and the ability to be recognized as a foreign agent or antigen. For anticancer therapy, microscopic loads are integrated into exosomes that are carried by them. They additionally serve in diagnostic purposes, inhibiting tumors, and immune therapy [13].
Another avenue for therapy is plant-based, specifically pomegranate. Secondary metabolites encountered in pomegranate extract, juice, and oil embody tannins and anthocyanin, which function as anti-inflammatory, antioxidant, and anti-proliferative substances. They also demonstrate anti-proliferative action by reprimanding the signaling pathways required for inhibition of tumors. Although it is a natural kind of medicine, it has no negative impacts, as opposed to many chemical drugs. As a result, they are potential chemopreventive or therapeutic agents [14]. Cannabis possesses the ability to inhibit proliferation of cells and signaling pathways. The cannabis plant has its own unique receptor ligand system including enzymes that aid in metabolizing functions. Non-psychoactive subclasses (components that are stored in the body after tetrahydrocannabinol metabolism) are employed in drugs manufacturing, which helps cancer patients curb their pain by inhibiting pathways such as MAPk and HIF-1α [15].
1.7 How phytochemicals influence signalling pathways
Understanding phytochemical-mediated signalling pathways is essential to understanding plant-derived chemicals’ biological effects. Phytochemicals affect cell proliferation, differentiation, and apoptosis via signalling pathways. Protein kinases, transcription factors, and other regulatory molecules in pathways are commonly modulated by these interactions. Most signalling pathways cause cancer or tumours. Plant-derived chemicals inhibit cancer in several ways [16]. One principal signalling cassette of the mitogen activated protein kinase (MAPK) pathway is the extracellular-signal-regulated kinase (ERK) pathway. Growth factors, hormones, and cellular stressors activate the ERK cascade to drive cellular activities as proliferation, differentiation, stress response, and others. MAPK/ERK promotes cell proliferation and differentiation, enabling oncogenic transformation and tumour progression [17]. Numerous phytochemicals have the capacity to regulate the dysregulated cascade of events within the MAPK signalling pathways [18] (Figure 1).
Figure 1. Plant Phytochemical, Their Secondary Metabolites and Their Role in Inibition of Signaling.
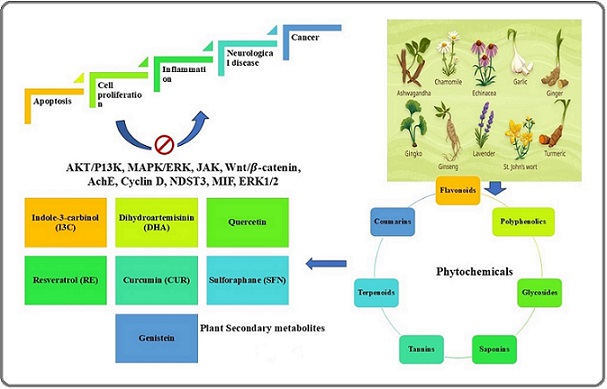
The EGFR is among the most extensively researched cell surface receptors in the medical field, due to its significance in cancer biology, developmental biology, and tissue homeostasis [19, 20]. The inhibition of the migratory capacity of squamous cell carcinoma cells by phytochemicals encompasses: the suppression of EGFR overexpression, the attenuation of extracellular signal-regulated kinase 1/2 (ERK1/2) protein activation and nuclear factor kappa (NF-κB) inactivation, and the reversal of epithelial-mesenchymal transition (EMT) [21] (Figure 2).
Figure 2. Depicts the Effect of Phytochemicals on Apoptotic Signaling Pathways. Pro-apoptotic proteins include caspases, Bax, Bak, Bid, Bad, and IkB, while anti-apoptotic proteins include Bcl-2, Bcl-XL, PI3K, Akt, IKK, and NF-kB. Plant phytochemicals such as resveratrol, Indole-3-carbinol, berberine, quercetin, and curcumin can inhibit anti-apoptotic proteins (Bcl-2) while stimulating pro-apoptotic ones (Bax, Bak); phytochemicals increase caspases; and apigenin inhibits NF-kB's anti-apoptotic action. Continuous blue lines indicate process stimulation (for simplicity, only the key apoptosis proteins are presented).
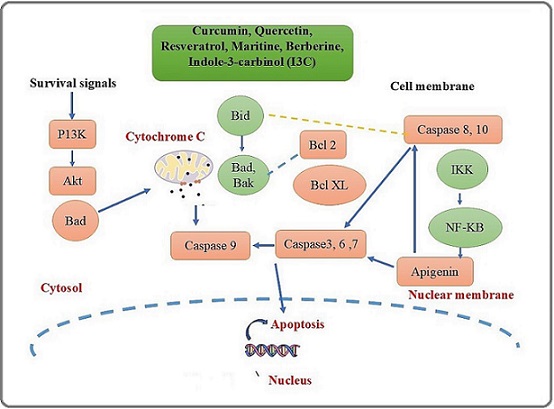
In addition to angiogenesis, neuropilins (non- tyrosine kinases receptors), tyrosine kinases receptors, immunodeficiencies, and integrins interact with VEGF signalling to cause cancer. Tumor growth can be reduced by tackling these facets. Many bioactive phytochemicals in natural products can suppress VEGF-promoting factors through signalling pathways, preventing cancer growth. VEGF signalling affects tumour cell activities and initiation in cancer cells via interacting with VEGF RTKs, NRPs, integrin, and other receptors. This connection activates signals that enhance cancer cell motility, adhesion, and proliferation. The formulation of innovative therapeutic strategies can be achieved by addressing and targeting the VEGF-associated boosting elements through the utilisation of either a singular agent or a combination of therapeutic compounds. Ilexgenin A, rosmarinic acid, and lupeol modulate proinflammatory cytokines, including TNF-α, to suppress VEGF and ultimately ameliorate cancer-related pain. They may serve as intriguing prospective medicines in anticancer therapy to alleviate cancer-related discomfort [22] (Figure 2).
2. Secondary metabolites
2.1 Plant secondary metabolites and their role as anti- cancer agents
Cancer continues to advance more rapidly in both industrialised and developing countries, despite the availability of certain effective treatments. However, long-term chemotherapies increase the likelihood of drug resistance, and off-site targeting renders this approach detrimental to patients’ health. So, scientists are attempting to utilise natural approaches to cope with the challenges. Recent biotechnology innovations, nanotechnological methods, and modifications to genomes have made cancer vaccine production and immunotherapy more feasible. Plant medication functionalized nano diamonds, together with nano-carriers such as gold and silver nano particles, are providing hope and more effective cancer therapy [23].
Advances in engineering, agriculture, and life sciences in recent years, together with the invention of CRISPR or Cas9 for gene technology, have honed the means for developing cancer drugs from plant-based sources. These approaches will allow genetic alterations in the plant genome, accumulation of important native medicinal proteins or compounds at one spot, and initiate the manufacture or recombinant proteins, including human antibodies, lectins, and vaccine candidates [24]. More than 3000 plants species have been demonstrated to be useful in combating cancer. In today’s world, over 60% of regularly used anticancer medications are derived from natural resources such as plants, aquatic organisms, and microbes. As of now, many plant-derived compounds have been uncovered and are now being investigated in clinical studies. These anticancer compounds were shown to be clinically effective against a wide range of cancer cells. Additional investigations in this area may lead to improved cancer treatment [25].
Plant secondary metabolites are widely and mainly classified into alkaloids, terpenes, tocopherols, phenols, flavonoids and cannabinoids (Figure 3).
Figure 3. Plant Secondary Metablites and Their Sources of Orgirin with Structural Formula.
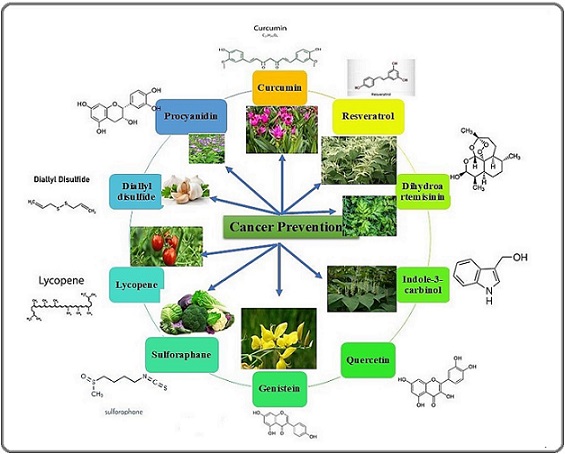
2.1.1 Alkaloids
Alkaloids are among the most diverse group of plant bioactive compounds. Thousands of alkaloids have been uncovered and are currently employed in various healthcare disciplines. Many of these compounds exemplify potent pharmacological effects. The first alkaloid isolations transpired during the nineteenth century, following the integration of several alkaloid- containing medications into medicine and coinciding with the discovery of the percolation method for drug extraction. Although the premise of alkaloid is relatively new, therapeutically effective chemicals have lately been discovered (early nineteenth century). Opium, a narcotic used for centuries for both analgesic and narcotic effects, was the first naturally occurring drug to be chemically identified. Plants, bacteria, aquatic species, and fungi all produce alkaloids, which are naturally occurring biological compounds containing nitrogen. They are categorized based on a variety of traits such as biological origin and chemical composition [26]. Alkaloids, which have a diverse spectrum of biochemical activity, are typically procured from Ranunculaceae, Papaveraceae, Fangke, Solanaceae, Apocynaceae, Rutaceae, Leguminosae, and Polygonaceae. Based on chemical classification, they can be classified into piperidine alkaloids, isoquinoline alkaloids, indole alkaloids, terpenoids alkaloids, steroidal alkaloids, quinoline alkaloids [27]. Plant alkaloids including morphine derivatives such as codeine, apomorphine, and narcotics (painkillers) utilized for the treatment of Parkinson’s disease, a form of papaverine (a muscle relaxant), and antibacterial compounds such as sanguinarine and berberine. Plant-based compounds have additionally been used to develop several potent anti-cancer drugs [26].
2.1.1.1 Bis-benzyl-isoquinoline alkaloids
Bisbenzylisoquinoline alkaloids are isolated from plant extracts that comprise mixtures involving multiple alkaloid classes with varying levels of structural complexity. Bis-benzylisoquinolines are typically found in combination with other isoquinoline alkaloid families such as benzylisoquinolines, morphinanes, berberines, and aporphines, all of which are derived from the same pharmacological origins [28].
2.1.1.2 Cinchona alkaloids
Malaria has been successfully combated using the bark of Cinchona trees and bushes endemic to Bolivia, Ecuador, and Peru. In 1820, Pelletier and Caventou identified the active component quinine (QN). Following this discovery, antimalarial treatment efficacy was improved by substitution QN bark with an unidentified alkaloid component. Botanists were also able to create Cinchona species with high levels of alkaloids deploying the quantitative method. Few alkaloids have lately isolated on an enormous scale. Cinchona bark has four main alkaloids: quinine (QN), quinidine (QD), cinchonidine (CD), and cinchonine (CN) [29].
2.1.1.3 Indole alkaloids
Moschamine is a feruloylserotonin, and serotonin is a widely understood neurotransmitter in the CNS derived from tryptophan. Moschamine-related indole alkaloids are a collection of natural compounds with distinct cinnamoyl groups.
2.1.1.4 Indole-3-carbinol (I3C)
Cruciferous plants including broccoli, cabbage, and cauliflower contain indole-3-carbinol (I3C), (Figure 3) which prevents cancer [30]. Its capacity to elicit G1 arrest of the cell cycle, induce apoptosis, and interfere with signal transduction pathways has been proven in a range of cancer cell lines, including prostate [31], melanoma [32], and breast cancer [33]. In the MDA- MB-231 breast cancer cell line, 3’-diindolylmethane, another I3C member, lessens angiogenesis, suppresses Akt/NF-кB signalling pathway activity and promotes apoptosis [30]. This compound has been shown to target multiple genes involved in cell cycle (cyclin D1, cyclin E, cyclin-dependent kinases CDK2, CDK4, and CDK6) and apoptosis (Bcl-2, Bcl-xl, survivin, suppressor of cell death protein IAP, x-chromosome associated IAP, pro-apoptotic gene Bax, induction of caspase-9 and caspase 3) [34].
2.1.2 Cannabinoids
Cannabinoids are a broad term that embodies a variety of chemicals that can modify the G-protein-coupled cannabinoid receptors (CBR), which are additionally recognized as cannabinoid receptor type-1 (CB1R) and cannabinoid receptor type-2 (CB2R), both of which are facets of the endocannabinoid system. They are classified as: (i) endogenous, (ii) synthetic, and (iii) phytocannabinoids [35].
2.1.2.1 Phytocannabinoids
Natural terpenoids or phenolic compounds derived from Cannabis sativa are termed as phytocannabinoids. Cannabimimetic ligands can act as CBR agonists or antagonists, or ECS enzyme inhibitors, in additional to their role in immune-mediated inflammations and infections, as well as nerve disorders such as neuro inflammatory disorders, neurodegenerative diseases, cancer, and autoimmune disorders [35].
2.1.2.2 Taxanes
Taxanes (e.g. Taxol/paclitaxel/albumin bound paclitaxel), oridonin, andrographolide, and diterpenes from coffee (e.g. cafestol, caffeic acid, and kahweol) have all been identified as potential anticancer diterpenes and derivatives. These diterpenes along with their derived compounds have been showed to be beneficial against an array of cancers, both human and nonhuman. Diterpenes are also known to protect biological systems from harmful substances that cause cytogenotoxic damage [36].
2.1.2.3 Resveratrol
Resveratrol, a terpenoids obtained from extract of grapes. Grapes (Vitis vinifera L.) are typical examples of fruits that are utilized not only for sustenance but additionally for specialized therapies due to their antibacterial, antioxidant, and anti-inflammatory properties. Grapes are high in phytochemicals, particularly resveratrol, a phytoalexin antioxidant found in red grapes that is both chemo preventive and therapeutic against a number of ailments. Resveratrol’s effects on breast, cervical, uterine, blood, kidney, liver, eye, bladder, thyroid, esophageal, prostate, brain, lung, skin, gastric, colon, head and neck, bone, ovarian, and cervical carcinoma have been investigated [37].
2.1.3 Flavonoids
Flavonoids, a key component of fruits, vegetables, cereals, barks, roots, stems, flowers, tea, and wine, contain a wide spectrum of useful phenolic substances. They play important functions in health maintenance, and subsequent research will uncover even more pertinent flavonoid components. Their primary functions include anti-oxidative characteristics, free radical scavenging, coronary heart disease prevention, hepatic inflammation protection, anti-inflammatory properties, as well as anti-tumor activity. They have an important function in provoking plant growth while alleviating oxidative stress [38]. Non flavonoids including luteonin, vanillic acid, erodictyol and protocatechuic acid have been remarkably reported as anti cancerous compounds (Figure 4).
Figure 4. Structural Representation of Some Non-flavonoid Compounds.
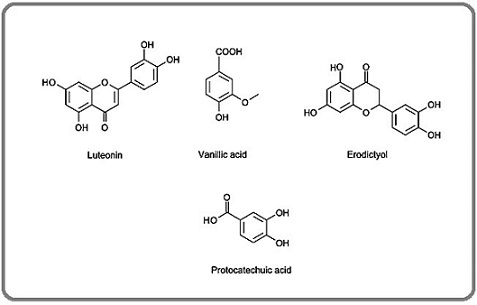
2.1.3.1 Quercetin
Quercetin inhibits heat shock protein and Ras proto-oncogene, down regulates mutant p53 in breast cancer cells, arrests the cell cycle in G1 phase [39], suppresses tyrosine kinase both in vitro and in vivo human studies [40], inhibits heat shock protein and Ras proto-oncogene [41]. Many of these effects have been shown in ovarian cancer cells. Heat shock protein-70 was found to be suppressed [42]. Cisplatin sensitises it to inhibit SK-OV-3 and CAOV3 ovarian cancer cell proliferation [43]. Kedhari et al [44] found quercetin inhibited PI3K, MAPK, and WNT pathways. Quercetin also inhibited melanoma cell survival and proliferation and caused JNK/P38 MAPK-mediated apoptosis [45] (Figure 2).
2.1.3.2 Kaempferol
Kaempferol, found in grapefruit, broccoli, and other plants, can, for example, regulate the activity of histone deacetylase (HDAC) in cancer cells. Tea’s epigallocatechin-3-gallate (EGCG) can influence the gene transcription of methyl transferases and noncoding RNAs in cancer cells. The most ubiquitous flavonoids in food are flavanols (3-hydroxylavones). The predominant plant flavanols are quercetin, myricetin, kaempferol, and fisetin, which can be found in a number of vegetables and fruits, including onions, apples, and strawberries [46].
2.1.4 Phenols
Phenols and polyphenolic substances are those with phenyl rings and hydroxyl substituents as important structural components, as addressed briefly in recent investigation. Plants may naturally synthesize phenols, which are widely distributed throughout the plant structure and are exclusively known as glycosides. Polyphenols are phytochemicals that have prospective health benefits. They are classed according to three primary factors: the amount of phenol rings present in the molecular structure, the types and quantity of functional groups, and the type of bond present between phenol rings. Flavanols, flavones, flavanones, isoflavones, anthocyanins, and non-flavonoid molecules are divided into subclasses based on these characteristics [47]. Non-flavonoid compounds comprise a number of acidic compounds including phenolic acids, hydroxycinnamic acids, lignans, stilbenes, and tannins. This group’s primary class consists of phenolic acids, specifically benzoic acid and cinnamic acid derivatives. Such compounds are seldom encountered in pure form, preferring to be conjugated with other polyphenols, glucose, quinic acid, or the plant’s natural structural components [48]. Recent investigations have demonstrated that phenol’s bioactivity is elevated by its own metabolites that developed in vivo and has an expanded array of biological activities such as anti-oxidation activity and anti-phlogistic action [47].
2.1.4.1 Resveratrol (RE)
RE a phytoalexin derived from grapes, red wine, peanuts, and berries, (Figure 3) is predominantly concentrated in Japanese knotweed (Polygonum cuspidatum) and serves as a potent antioxidant. RE disrupts AKT activity and induces apoptosis [49], in addition to also promoting p53 acetylation and apoptosis [50]. Resveratrol inhibits proliferation, migration, and invasion through the NEAT1-mediated Wnt/β-catenin signalling pathway [51].
2.1.4.2 Phenolic amides
Polyphenols may have an amide group. Avenanthramides and capsaicinoids are believed to be found to have antioxidant and anti-inflammatory properties. Capsaicinoids can be noticed in jalapeño peppers and avenanthramides in oat products. As a result, interest in these compounds has grown in recent years.
2.1.4.3 Curcumin (CUR)
CUR a derivative of turmeric, is derived from the roots of the Curcuma longa. Howells et al. identified various bioactivities of this chemical (Figure 3). CUR inhibits cyclin D1, cyclin E, and MDM2, while activating the tumor suppressors p21, p27, and p53 [52, 53]. CUR promotes apoptosis by inhibiting the PI3K/AKT pathway [54].
2.1.5 Tocopherols
Tocopherols naturally contain a chromanol ring and phytyl side chains. It is found in vitamin E, and the number of phytyl side chains dictates its natural form. It includes tocopherols (RRR) α, β, γ, δ and tocotrienols that are αTE, δTE, βTE, and others. Tocotrienols are more unsaturated than tocopherols owing to they have three double bonds while tocopherols only have one. This is due to the fact that it consists of a phenolic group connected to a chromanol ring, which releases a hydrogen atom that functions as a lipid peroxide radical scavenger; all sorts of vitamin E are lipophilic antioxidants. Plants naturally produce vitamin E, which is prevalent in nuts, seeds, and plant oils [55]. Several epidemiological studies have investigated an alleged link between vitamin E and cancer risk. Vitamin E’s cancer-fighting abilities have been linked to anti-oxidant, anti-inflammatory, anti-proliferative, anti-angiogenic, immunological modulatory, and HMG CoA reductase enzyme inhibition pathways [56].
2.1.6 Isoflavones
Genistein, an isoflavone found predominantly in soybeans, influences many signalling pathways in cancer cells (Figure 3). This bioactive substance regulates the cell cycle and apoptosis of prostate, breast, and lung cancer cell lines by blocking the NF-kB and Akt signalling pathways, as well as promoting G2/M and G0/G1 arrest in multiple cancer cell lines [57]. Genistein hampers anti-apoptotic proteins BcL-XL and BcL-2 while driving pro-apoptotic proteins Bax, Bak, and Bad [57].
2.1.7 Organosulfur
2.1.7.1 Sulforaphane (SFN)
Cauliflower, broccoli, and cruciferous vegetables have high levels of SFN (Figure 3). SFN inhibits tumour angiogenesis by reduced cell survival, migration, and developing tubes in HUVEC-epithelial cell lines, potentially through impeding STAT3/HIF-1α/VEGF signalling [58]. It was recently demonstrated to inhibit YAP1 signalling, resulting in reduced cancer stem cell survival and tumour proliferation in epidermal squamous cell carcinoma [59].
2.1.7.2 Diallyl disulfide (DS)
DS naturally occurring in cauliflower, broccoli, garlic, and cabbage (Figure 3), has been indicated to enhance G2/M cell cycle arrest in cancer cells by producing ROS and repressing HDAC activity [60].
2.1.8 Carotenoids
Tomatoes contain an abundant quantity of lycopene, a carotenoid derived from vegetables and fruits (Figure 3). Lycopene decreases intercellular reactive oxygen species (ROS) via increasing the activity of antioxidants such as glutathione-S-transferase-omega-1 and superoxide dismutase-1 (SOD-1), as well as ERO-1. Lycopene has been illustrated to inhibit the growth of ovarian tumors [61], lessen the risk of breast and prostate tumors, and substantially limit the cellular growth of colorectal [62] and lung cancer [63].
2.3.1 Alkaloids activity
2.3.1.1 Matrine
Matrine is a naturally occurring alkaloid uncovered in the roots and stems of the Sophora legume plant. It has been demonstrated to inhibit proliferation and pro-apoptotic in a range of carcinomas. According to functional studies, matrine treatment increased mitochondrial dysfunction and apoptosis by decreasing protective mitophagy. Matrine’s pro-apoptotic effects on HepG2 cells were mitigated when mitophagy was reinstated. Matrine modulates mitophagy through the PINK1/Parkin pathways, according to molecular study. Matrine dwindled mitophagy by inhibiting the PINK1/ Parkin pathways, while activating the PINK1/Parkin pathways increased mitophagy activity and HepG2 cell survival. Matrine promoted HepG2 cell death via a novel mechanism that blocked mitophagy and the PINK1/Parkin pathways [64] (Table 1).
Plant derived compound | Prevention of cancer type | Target sites | References |
Matrine | Hepatocellular carcinoma | Inhibit Mitophagy, Inhibit PINK1/Parkin pathways | [66] |
Vinca alkaloids | Breast, ovarian, colon and lung cancers | topoisomerase (TOPO)↓, kinesin Eg5↓, telomerase ↓, cyclin dependent kinase (CDK) ↓, IκB kinase (IKK) ↓, polo-like kinase-1 (PLK1) ↓ | [67] |
Berberine | Ovarian cancer | Suppress caspase-dependent pathway, Inhibit RIPK3–MLKL pathway | [68] |
Baicalin | Colon cancer | Ras/Raf/MEK/ERK↑, p16INK4A/Rb ↑, DEPP ↑ | [70] |
Gossypin | Prostate cancer | NFKβ1 inhibition, CASP3, CASP9 ↑ | [71] |
Ginsenoside | Thyroid cancer, Prostate cancer | Rho GTPase ↓, Rac-1 ↓, Cdc42 protein ↓, VEGF-C ↓, VEGF-A ↓ | [72] |
Cucurbitacin | Pancreas cancer | AFAP-AS1 ↓ | [73] |
Plumbagin | Prostate cancer | EGFR ↓, NFKβ ↓ | [75] |
2.3.1.2 Vinca alkaloid
Vinca alkaloids, inhibits topoisomerase (TOPO), kinesin Eg5, telomerase, cyclin dependent kinase (CDK), Iβ kinase (IKK), and polo-like kinase-1 (PLK1) in the evolutionary phases of the cell cycle (Figure 5), as a class of potential carboline derivatives, rising data has shown its auspicious functions in combating cancer [65] (Table 1).
Figure 5. Chemotherapeutic Drugs that Target Cell at Different Stages and their Mechanism of Action Performed in Cell Cycle.
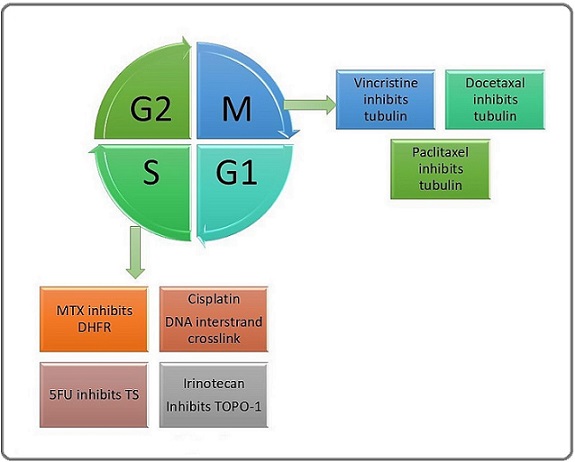
2.3.1.3 Berberine
Recently, researchers investigated the combined effects of BBR and DDP on OVCAR3 and patient-derived primary ovarian cancer cell lines. Findings showcased that combining BBR and DDP treatment considerably improved DDP’s anti-tumor activity by eliciting apoptosis and necroptosis in ovarian cancer cells. The caspase- dependent route has been implicated in apoptosis, whereas the RIPK3-MLKL pathway was pertinent in necroptosis. BBR in combination with DDP considerably reduced cell growth, implying that it could be employed as a potential therapeutic strategy for the management of ovarian carcinoma in the health care facility [66] (Table 1).
2.3.2 Flavonoids activity
Recent research findings on flavonoids have uncovered their extensive biological properties, which may involve modulating enzymes involved in ROS scavenging, participating in cell cycle arrest, initiating apoptosis and autophagy, and thwarting tumour cell progression and cancer transformation into metastatic tumour. Flavonoids played a multifaceted function, acting as anti-oxidants for healthy cells and powerful pro-oxidant agents for cancerous cells, triggering apoptotic cell death and inhibiting pro-inflammatory signalling pathways [67] (Figure 5).
2.3.2.1 Baicalin
Baicalin, a naturally occurring glycosidic flavonoid, exhibits cancer-preventing and antioxidant activities in many malignancies. Baicalin increased DEPP synthesis in HCT116 colon cancer cells besides regulating many signalling pathways such as Ras/Raf/MEK/ERK and p16INK4A/Rb. In this interim period, these activities occur as a result of baicalin’s antioxidant activity. In HCT116 cells, increased activity of senescence- associated-galactosidase (SA-β-Gal) and overexpression of DEPP were mediated by the aforementioned signalling pathways. Cell cycle arrest, apoptotic cell death, and Baicalin-mediated suppression of growth were all negated in colon cancer cells by inhibiting DEPP by RNAi. Researchers furthermore, established that baicalin therapy significantly suppressed cancer progression in a xenograft mice model of human colon cancer, that was associated with the stimulation of cancer’s cellular death via overexpression of DEPP and stimulation of Ras/Raf/MEK/ ERK signaling procedures in vivo. They observed that, in addition to baicalin treatment, the hypoxia-response protein DEPP functions as an up regulator, directing the signalling cascade and averting human colon cancer using anti-oxidative drugs such as curcumin and sulforaphane, resulting in cancer cell death. Such findings imply that by serving as an antioxidant, baicalin upregulates DEPP expression and activates its downstream Ras/Raf/MEK/ ERK and p16INK4A/Rb pathways, leading to death of colon cancer cells [68] (Table 1).
2.3.2.2 Gossypin
Gossypin demonstrated an inhibitory effect on the PC3 cell line that varied with time and dose. Gossypin substantially raised apoptotic genes (CASP3, CASP9) and decreased NFKβ1, which suppresses cell death (similar to cisplatin). Gossypin 50 and 100 µM significantly induced apoptosis in PC-3 cells [69] (Table 1).
2.3.2.3 Ginsenoside
Ginsenoside Rg3, a bioactive component of Panax ginseng, is a popular traditional Chinese medicine deployed as a tonic around the world. It also prevents tumour spread, thus rendering it a potent anticancer drug. Rh2, Rg1, Rg3, Rk1, F2, and Rd are ginsenosides with anticancer activity. Rh2, Rg1, Rg3, and Rd ginsenosides have been outlined to alleviate tumour metastasis. Ginsenoside Rg3 showed strong antimetastatic activity in several malignancies by inhibiting migration, invasion, and angiogenesis. Rg3 inhibited Rho GTPase, averting lamellipodia growth and elevating microspike production in thyroid cancer cells. Rac-1 and Cdc42 protein levels were reduced by Rg3. Some of major functions were shown in PTC and ATC cancer cells by the Rg3 that are inhibition of matrix proteins (MMP-2, MMP-9) in type 4 thyroid cancer cells. In PTC cancer cells, VEGF C is suppressed, whereas VEGF A undergoes suppression in ATC cells. Inhibited CD31 staining has convincingly demonstrated that Rg3 may limit the dissemination of PTC to the lymphatic system and ATC angiogenesis [70] (Table).
2.3.3 Terpenoids activity
2.3.3.1 Cucurbitacin
Cucurbitacin B (CuB) is a naturally occurring tetracyclic triterpene that has anticancer properties against a variety of tumour types. CuB’s anti-pancreatic cancer activity was studied by suppressing the expression of actin filament associated protein 1-antisense RNA 1 (AFAP1-AS1), a cancer-related long noncoding RNA. CuB reduced the expression of AFAP1-AS1 in pancreatic cancer (PC) cells, directing them to enter the G2/M cell cycle. Endogenous AFAP1-AS1 expression was reduced, increasing the availability of miR-146b-5p in PC, whereas miR-146b-5p overexpression oppressed endogenous AFAP1-AS1 expression [71].
2.3.3.2 Gypenosides
Cell proliferation, apoptotic cell death and cell cycle inhibition at G0/G1 phases has been the major actions performed by Gypenosides in the A549 cells. There are 9 major potential targets of gypenosides i.e. STAT3, VEGFA, EGFR, MMP9, IL2, TYMS, FGF2, HPSE, LGALS3. It has more profound effects on 17 main metabolism-related proteins, resulting in altered synthesis of metabolites such as uridine 5’-monophosphate and D-4’-Phosphopantothenate, as well as changes in their metabolic pathways, which include pyrimidine metabolism, pantothenate, and CoA biosynthesis. Western blotting, an electrophoretic approach, determined that gypenoside-induced suppression of A549 cells via MMP9, STAT3, and TYMS ported an indirect effect on the pyrimidine absorption, pantothenate, and CoA biosynthesis pathways [72].
2.3.3.3 Plumbagin
A medicinal plant. Plumbago zeylanica L exhibits a substantial amount of quinoid compounds in its roots, one of which is plumbagin (PL), as evidenced by in vitro and in vivo experiments. It inhibits the progression of PC cells. When PC cells were exposed to PL treatment, PANC1, BxPC3, ASPC1 cells viability was suppressed and apoptotic cell death was induced by PL. This treatment causes (1) suppression of EGFR, pStat3Tyr705 and pStat3Ser727, (2) inhibits binding of Stat3 with DNA, (3) reduces the interaction of Stat3 and EGFR especially for xenograft cells as well as cultured PC cells (PANC1). PL reduced both NF-κB phosphorylation and DNA binding activity. Downstream target genes of these pathways were likewise subjugated, including cyclin D1, MMP9, Survivin, and others. All of these findings indicated that PL could be a potential treatment for human PC cells [73].
2.3.3.4 Dihydroartemisinin (DHA)
DHA is a derivative of artemisinin, a chemical derived from the daisy-family wormwood Artemisia annua and used by ancient people (Figure 3). Through apoptosis, it kills various cancer cells. It is cytotoxic to papilloma virus-expressing epithelial cells in vivo and in vitro [74]
and induces apoptosis by mitochondrial caspase pathway activation without p53. It mitigates RPMI18226 multiple myeloma cell VEGF expression [75]. and blocks HUVE cell proliferation, migration, and tube formation. It exhibits cytotoxic effects on C6 glioma cells and suppresses the activation of hypoxia-inducible factor-1 alpha [76]. DHA has demonstrated a synergistic impact with temozolomide, inducing lethal effects in rat C6 glioma cells [76]. It has been demonstrated to attach to human fortillin, an anti-apoptotic protein that is overexpressed in numerous malignancies, therefore enhancing its degradation and desolation.
3. Phytochemical-based nano-drug delivery systems (Pb-NNDS)
Herbal drugs have limited clinical use due to their active components’ weak solubility and hydrophobicity, which decreases bioavailability and increases systemic clearance, necessitating repeated administration or higher doses. Phenolic phytoconstituents are unstable in experiments but have excellent antioxidant capacity. Despite their potential, bioactive chemicals like paclitaxel and curcumin have low solubility and bioavailability, requiring toxic solvents. Nano or micro formulations may address this. Nanocarriers or sustained-release dosage forms use polymer or lipid carriers to administer medications transdermally, buccally, or orally, and parenterally. They improve treatment efficacy and target localization, which maximizes patient compliance [77].
Polymeric nanoparticles (synthetic and natural) for delivering phyto-derived medicinal medicines like curcumin, Epigallocatechin-3-gallate (EGCG), berberine, chrysin, and quercetin are being studied worldwide. Kumari et al. [78] created a formulation of curcumin nanoparticles and PGMD (poly-glycerol-malic acid-dodecanedioic acid) to combat breast cancer cells [78]. Both nanoparticles demonstrated an entrapment efficiency of 78% to 81%.
Zeng et al. used nanotechnology to boost the ability of EGCG to target MCF-7 cells. Two distinct forms of EGCG nanoparticles (FA-NPS-PEG and FA-PEG-NPS) were created, and their characteristics and effects on MCF-7 cells were studied. The findings revealed that EGCG nanoparticles are both extremely resilient and stable [79]. These nanoparticles surpassed EGCG in terms of cellular absorption, suppression of MCF-7 cell proliferation, and altering the expression of numerous key PI3K-Akt pathway regulatory proteins.
Solanki et al. employed the dissolution technique to encapsulate berberine in bovine serum albumin nanoparticles, (BSA NPs). An apoptotic and cellular uptake research determined that BBR-BSA NPs were more detrimental to MDA-MB-231 breast cancer cells. However, higher intracellular absorption findings indicate that berberine-BSA NPs might substantially boost anticancer activity at a lower berberine dose [80].
2.2 Multidrug resistance
Cancer-stricken individuals become more resistant to prospective cancer therapies because after prolonged administration, the body becomes accustomed to the drug and fails to respond to it. Drugs that were previously presumed to have favorable effects are now causing negative side effects, which poses a more critical scenario. This disease is known as multidrug resistance (MDR), which develops as a result of long-term chemotherapy and suppression or hindered apoptotic death of cancer cells. To address this, anti-cancer medications foster autophagy. It promotes apoptosis in multidrug resistant cells [81]. This condition is multifaceted, since it integrates host factors (position of the tumor, environment around the tumor), tumor variables (benign or malignant), and host-tumor connections [82].
Chemotherapeutic drugs can be classified into subcategories based on their mode of action, which include antimetabolites, alkylating compounds, mitotic spindle blockers, topoisomerase inhibitors, and others. Individuals on standard chemotherapeutics coupled with innovative targeted medications have a 90% risk of death from cancer as a result of multidrug resistance. Major factors behind MDR include enhanced drug metabolism, accelerated biotransformation and transportation of medicines, fluctuations in growth hormones, an enhanced capacity to repair DNA, and genetic variations such as gene mutations, amplifications, and epigenetic modifications [83].
MDR cancer cells undergo significant changes in their plasma membrane. They comprise a significant number of carbohydrates and lipids, including cholesterol, as well as a high concentration of long chain saturated fatty acids. MDR cells also produce a unique lipid membrane enclosing their internal organelles, thereby reducing the availability of oxidizable fatty acids [84]. Some cells are MDR due to the fact that they embody pathogenic transporters. Their elevated expression causes the cells to become more resistant. ABC transporters, also known as ATP binding cassettes, are an excellent instance of transporters [85]. Almost hundred drugs have been approved for cancer treatment, but they have adverse outcomes due to offsite toxic impact. Quinolone and Isatin metabolites and subclasses have demonstrated more selective activity along with substantial therapeutic benefits on MDR cancer cells [86]. Furthermore, comprehensive investigations of ABC transporters have documented their dual role, since they develop MDR; nevertheless, some ABC transporters can be exploited to develop medicines or modulators for MDR inhibition [87].
The MDR phenotype is resistant to a wide range of anti-tumor medications, each having a distinct mechanism. MDR is facilitated by an assortment of pathways, including host factors, tumour factors, and tumor-host interactions. Host variables include genetic differences and pharmacological interactions. The primary cause of reduced drug absorption was defective influx transporters. Increased drug efflux occurs primarily by the overexpression of MDR efflux transporters, which belong to the ATP-binding cassette superfamily. Drug efflux is achieved by extracellular vesicles (EVs) or drug-loaded lysosomes that undergo exocytosis, inhibition of cell death mechanisms (i.e., anti-apoptotic modalities), enhanced DNA repair mechanisms, environmental or genetic alterations, and microRNA suppression. Cancer stem cell plasticity, coupled with intra-tumor heterogeneity and dynamics, are critical tumour drivers. Examples of tumor-host interactions include:
• The tumor microenvironment’s function
• The selective pressure of various stressor conditions and agents
• Acidic pH
• EVs mediate the intracellular transfer of characteristics.
The engagement of these several elements in MDR emphasizes the significance of personalised medicine and real-time tailored therapy for individual cancer patients [88].
The likelihood of cancer developing resistance to chemotherapy (MDR) remains a significant barrier to effective cancer treatment. Overexpressed membrane- embedded drug transporters are the leading cause of multidrug resistance (MDR). P-glycoprotein (P-GP), MDR1/ABCB1, MRP1/ABCC1, MRP2, and breast cancer resistance proteins (BCRP/ABCG2) are thought to be major contributors to the development of MDR. The current study examines several groups of natural products (flavonoids, alkaloids, terpenoids, and coumarins) as prospective MDR modulators and as a source of intriguing lead compounds (from plants, marine species, and microbes). Tetrandrine, a bisbenzyl isoquinoline alkaloid derived from the Chinese herb Stephania tetrandra (Han-Fang-Chi), has recently been tested in clinical studies for its MDR reversal efficacy [89].
Numerous studies have connected drug resistance to inappropriate expression of long non-coding RNAs (lncRNAs). In the past few years, lncRNAs have received a great deal of interest in related fields. Previous studies has shown that lncRNAs play a substantial part in a range of tumours due to their distinct modes of action. LncRNAs modulated miRNA, signalling pathways, proteins, cancer stem cells, pro- and anti-apoptosis, and autophagy, all of which contributed to cancer medication resistance. lncRNAs can be utilized to pinpoint prospective therapeutic targets in chemotherapy, which may lead to future remedies to the MDR [90].
Resistance mechanisms might vary between cancer types. According to the most recent developments in genetic studies, imaging, and novel delivery methods, we could potentially able to define and circumvent resistance, for example, by blocking the exact target at the tumour site. As consequence, combined pharmaceutical treatments, in which one therapy reverses or blocks resistance amplification while another treatment targets the cancer cell, are effective. Drug exposure varies greatly depending on the drug’s physicochemical properties. In this context, multi-compound nanoparticles represent a terrific method to optimise therapeutic dosage with a single physicochemical profile. Nanoparticles have transformed from general-purpose, non-targeted monodrug transporters to sophisticated multidrug, sequence, and time-controlled drug distribution systems. Furthermore, nanoparticles can be tailored to efficiently deliver drugs to cancer cells while simultaneously tackling existing resistance mechanisms. Complex resistance mechanisms, that require sequence- specific route blockage to triumph over drug resistance, can also be perturbed. This will pave the way for the development of highly efficient, multifunctional, and personalised novel therapeutic Nanomedicines. As previously noted, several ABC efflux pumps, including as MRPs and BCRP, are elevated in a range of malignancies and are frequently involved in imparting resistance to an array of oncological drugs. Several nanoparticle-based techniques to address these resistance mechanisms have been published. Combining cytostatic drugs and efflux pump inhibitors appears to enormously strengthen therapeutic efficacy [91].
4. Comparative analysis between plant based therapies and conventional treatments
The incorporation of plant-based remedies into conventional medical practice reflects a rapidly growing sector of healthcare that blends the best of both traditional and modern approaches to medicine. Plant-based therapies, frequently originating from herbal medicine, have been utilised for thousands of years to treat a wide range of disorders throughout cultures. As interest in natural and integrative medicine escalates, there is a great opportunity to incorporate these therapies into traditional medical practices to provide comprehensive and effective care. Plant-based therapies refer to a wide range of treatments which employ natural plant extracts and chemicals. These therapies are used to manage and treat an array of diseases, including infection, inflammation, chronic diseases, and mental health issues. Plant active chemicals such as alkaloids, flavonoids, and terpenes have been proven to have a wide range of pharmacological actions, including anti-inflammatory, antioxidant, and antibacterial characteristics.
Many plant-based medicines have minimal detrimental impacts than synthetic pharmaceuticals, making them ideal for prolonged use. Evidence-based techniques prove essential for effectively integrating plant-based medicines into mainstream medical practice. This involves executing comprehensive scientific evaluations of the safety, effectiveness, and mechanisms of action of these medicines. Conducting clinical trials to figure out the efficacy of plant-based treatments in treating specific ailments. To ensure the safety and efficacy of plant-based treatments, they must be compounded and administered systematically. This includes standardizing the active components and ensuring the substances’ purity. Figuring out how plant-based substances interact with the body on a molecular level aids in discovering possible therapeutic targets and foreseeing drug interactions.
5. Limitations and future perspectives of plants based cancer treatment
5.1 Limitations
Following research investigations, developments in pharmaceuticals and plant biotechnologies have made the treatment of different kinds of tumours increasingly efficient. However, in addition to their efficiency, they have significant adverse effects.
Vinca alkaloids, like taxanes and Thalidomide, elicit serious adverse impacts on the body owing to offsite toxicity. They undermine peripheral sensory, motor, and autonomic neurons, resulting in chemical-induced peripheral nephropathy. Taxanes are fatal because of their neurotoxic effects [92]. Quercetin is a phytochemical exhibiting anti-tumor potential, however its low solubility, fast metabolism, biotransformation, and elimination impede its therapeutic value. For the drugs to have a greater effect, it must be routed simultaneously with two or more chemical components [93].
Irinotecan, a camptothecin derivative, has been portrayed to be beneficial against cancer progression because it inhibits topoisomerase 1 in the lungs, breast, pancreas, colon, and many other malignancies. However, its limited survivability and absorption limit its effectiveness as an anticancer drug. The main negative effects include dysbiosis and intestinal poisoning [94]. Polyphenols are additionally limited by their low solubility, substantial metabolism, and quick elimination from the body. These factors might influence the effective dose and could also have minimal impact on tumour cells as well [95]. Nano-carriers are also employed to transport drugs to the target region, however their instability and low drug loading capacity render them ineffective for cancer treatment. For the sake of high efficiency more research and data collection is needed.
5.2 Strategies to overcome the challenges associated with plant-based treatment
According to the few information stated above, plant- based cancer treatment has both prospective impacts and off-site harm on the body. Their limited bioavailability, low absorption, and quick metabolism all have detrimental effects on their roles. To address this issue, far more efficient technologies must be implemented to improve their effectiveness. DNA-based technologies, liposomal analysis, microarray technologies, nanomedicines, and nanoparticles showcased more efficiency, and future research will lead to outstanding treatment alternatives for patients. Furthermore, phytochemicals delivered through radiation or chemical techniques have an enhanced efficiency rate.
5.3 Future perspective and integrating phytochemicals with nanomedicine
As time passes, new findings about plant-based medicines and their mechanisms of action on various types of malignancies are being investigated. New approaches to addressing this life-threatening condition are being investigated, and more understanding and research will be required. Along with chemotherapies, radiotherapies, hormone therapies, and phytochemical drugs, nano carriers and nanomedicines are emerging technologies that will aid in the treatment of cancer. Platinum-based nanocarriers are the most tailored and credible for targeting tumours at specific sites, with a lower risk of offsite injury.
As tumour genesis and progression pathways are more understood, combination medication therapy has shown promise for cancer treatment. Nanotechnology advancements open up new avenues of application. Emerging tactics and methodologies aid for creating nano drug delivery systems (NDDSs) for codelivery of plant active components and chemotherapeutic medicines in order to overcome delivery hurdles. NDDSs must overcome all of these obstacles before they may exhibit successful and effective anticancer action. Any challenges with these delivery systems could result in cancer treatment failure. To overcome these limitations, NDDSs should be developed specifically for the physiological processes in the body. However, the synthesis of multifaceted and intelligent tumor-targeted NDDSs is exceedingly challenging, lessening the drugability of NDDSs. However, the synthesis of multifaceted and intelligent tumor-targeted NDDSs is exceedingly challenging, lessening the drugability of NDDSs. NDDSs for codelivery continue to encounter significant problems in terms of formulation, design, synthesis, and evaluation. Additional investigation is needed to better understand the pathogenic aspects of cancers. Further research and analysis of past data will lead to the identification of an obscured mechanism for such techniques, which will be adequate to cure this disease and lower the likelihood of cancer occurrence.
In conclusion, cancer is typically characterised as abnormal cell growth or division. Immune impairment is a critical component that contributes significantly to cancer progression. This devastating illness is expanding worldwide, with about 14 million people diagnosed with cancer each year. Obesity has been linked to over 13 different types of cancer, including multiple myelomas, gallbladder, colorectal, and hepatocellular carcinoma. Cancer cells advance through a variety of signaling pathways. These pathways may differ across different types of cancerous tumours. Different therapeutic techniques, such as chemotherapy, radiation, immunological therapies, and hormone therapies, are used in healthcare. Treatment devastating events caused by the microenvironment surrounding the tumour or cancer cells. Previous research has revealed that plants’ natural bioactive components are exceedingly effective against cancer therapies. They have anti-cancer and immunological modifying capabilities that can trigger a cell-mediated immune response in the body. This review highlights the anti-proliferative, anti-inflammatory activities of phytochemicals that can cure cancer. Alkaloids, cannabinoids, phenols & polyphenols, terpenes, tocopherols and flavonoids are the major categories. Alkaloids such as Berberine, Matrine, Vinca alkaloids are used while flavonoids containing compounds are Gossypin, Gypenosides, Ginsenosides, etc. They primarily inhibit mitophagy and disrupt Ras/ Raf/MAPK, EGFR, VEGF, and other signaling pathways, resulting in cell cycle arrest at various stages of the cell cycle. Extensive research and past information have demonstrated that, in addition to their helpful advantages, they also have adverse consequences. Additional strategic methods and research must be developed to overcome phytochemical-based cancer treatment.
Institutional approval
This study does not have any ethical subjects, therefore do not need any ethical approval.
Acknowledgments
Statement of Transparency and Principals:
Funding acquisition
No Govt or private funding was received for this study.
Data availability
Not Applicable
CRediT authorship contribution statement All listed authors must have made a significant scientific contribution to the research in the manuscript, approved its claims, and agreed to be an author. Zunaira Batool: Writing – original draft, Data curation, Conceptualization. Shumaila Kiran, Amina Arif, Adil Jamal, Muhammad Naveed Shahid: Writing – review & final editing, Supervision, Conceptualization, Methodology.
Declaration of competing interest
The authors declare that they have no known competing financial interests or personal relationships that could have appeared to influence the work reported in this paper.
List of abbreviations
EGFR, Epidermal Growth Factor Receptor; NADPH, Nicotinamide Adenine Dinucleotide Phosphate; MAPK, Mitogen activated protein kinase; NP’s, Nanoparticles MDR, Multidrug resistance; ABC transporter, ATP-binding cassette transporter; ATP, Adenosine triphosphate; NRF2, Nuclear factor erythroid 2-related factor 2; SOX4, Box transcription factor 4; YAP, Yes-associated protein; TAZ, Transcriptional Coactivator with a PDZ-binding motif; TGFβ, Transforming growth factor β; KRAS, Kristen rat sarcoma viral oncogene; RAF-1, Rapidly accelerated fibro sarcoma 1; NFkβ, Nuclear factor kappa-light- chain-enhancer of B cells; HDAC, Histone deacetylase; EGCG, Epigallocatechin-3-gallate; EV, Extracellular vesicles; LncRNA, Long non-coding RNA; miRNA, micro-RNA; MRP’s, Multidrug resistance associated protein; BCRP, Breast cancer resistance protein; APEX1, DNA lyase encoding gene; BRCA, Breast cancer gene 1; XRCC, X-ray repair cross complementing 2; ATM, Ataxia Telangiectasia mutated; CHEK2, Checkpoint kinase 2; PALB2, Partner and localizer of breast cancer 2; RAD51, DNA repair protein encoding gene, XPD; X-ray photoelectron diffraction; MDR1, Multidrug resistance mutation 1; ABCB1, ATP-binding cassette transporter protein B1; MRP1, Multidrug resistance protein 1; ABCC1, ATP binding cassette subfamily C member 1; BCRP, Breast cancer resistance protein; ABCG2, Breast cancer resistance protein; TalaA, Talaroconvolutin A; CRC, Colorectal cancer; GSS, Glutathione synthetase; HepG2 cells, Human Liver Cancer Cell line; PINK1, Parkin pathways; BBR, Beberine; DDP, Di-ammine-di- chloro-platinum; OVCAR3, Ovarian cancer cell lines; MLKL, Mixed lineage kinase domain like protein; RIPK3, Receptor interacting serine/threonine kinase 3; ROS, Reactive oxygen species; IKK, Inhibitor of Kappa B Kinase; HCT116, Human Colon Cancer Cell Lines; DEPP, Decidual protein induced by progesterone; Ras, Rat Sarcoma Viral; MEK, Mitogen-activated protein kinase kinase; ERK, Extracellular-signal-regulated kinase; Rb, Retinoblastoma protein; CASP 3, Caspase 3 Protein; CASP 9, Caspase 9 Protein; ATC, Anaplastic thyroid cancer; PTC, Papillary thyroid carcinoma; VEGF, Vascular Epidermal Growth Factor; MMP 2, Matrix Metalloproteinase 2; MMP 9, Matrix Metalloproteinase 9; CD31, Cluster Differentiation 31; AFAP1-AS1, Actin filament associated protein 1-antisense RNA 1; STAT3, Signal transducer and activator of transcription 3; IL2, Interleukin-2; TYMS, Thymidylate Synthetase; FGF2, Fibroblast growth factor; HPSE, Human Prostate-Specific Ets; LGALS3, Galectin 3 Protein; A549 Cells, Human Lung Cancer Cells; PANC1 Cells, Human Pancreatic Cell Lines; BxPC3 Cells, Human Pancreatic Cancer Cell Line; ASPC1 Cells, Pancreatic Cell Lines; CDK, Cyclin-dependent kinases
References
- Cancer and cure: A critical analysis Roy P. S., Saikia B. J.. Indian Journal of Cancer.2016;53(3). CrossRef
- Global Cancer Statistics 2020: GLOBOCAN Estimates of Incidence and Mortality Worldwide for 36 Cancers in 185 Countries Sung H, Ferlay J, Siegel RL , Laversanne M, Soerjomataram I, Jemal A, Bray F. CA: a cancer journal for clinicians.2021;71(3). CrossRef
- Exploring the Burden of Cancer in Pakistan: An Analysis of 2019 Data Tufail M, Wu C. Journal of Epidemiology and Global Health.2023;13(2). CrossRef
- Anticancer Activities of Plant Secondary Metabolites: Rice Callus Suspension Culture as a New Paradigm Ramakrishna W, Kumari A, Rahman N, Mandave P. Rice Science.2021;28(1). CrossRef
- The anti-inflammatory and antioxidant activity of 25 plant species used traditionally to treat pain in southern African Adebayo SA , Dzoyem JP , Shai LJ , Eloff JN . BMC complementary and alternative medicine.2015;15. CrossRef
- Synergistic Anticancer Activities of Natural Substances in Human Hepatocellular Carcinoma Kojima-Yuasa A, Huang X, Matsui-Yuasa I. Diseases (Basel, Switzerland).2015;3(4). CrossRef
- Food Plant Secondary Metabolites Antiviral Activity and Their Possible Roles in SARS-CoV-2 Treatment: An Overview Giordano D, Facchiano A, Carbone V. Molecules (Basel, Switzerland).2023;28(6). CrossRef
- Types of Cancers Prevailing in Pakistan and their Management Evaluation Tariq A, Majeed I, Khurshid A. Asian Pacific journal of cancer prevention: APJCP.2015;16(9). CrossRef
- Cancer in Pregnancy McCormick A, Peterson E. Obstetrics and Gynecology Clinics of North America.2018;45(2). CrossRef
- Oxidative Stress in Cancer Hayes JD , Dinkova-Kostova AT , Tew KD . Cancer Cell.2020;38(2). CrossRef
- Tumor microenvironment: recent advances in various cancer treatments Wang J.-J., Lei K.-F., Han F.. European Review for Medical and Pharmacological Sciences.2018;22(12). CrossRef
- Gold Nanoparticles in Diagnostics and Therapeutics for Human Cancer Singh P, Pandit S, Mokkapati V. R. S. S., Garg A, Ravikumar V, Mijakovic I. International Journal of Molecular Sciences.2018;19(7). CrossRef
- Exosome-based immunotherapy: a promising approach for cancer treatment Xu Z, Zeng S, Gong Z, Yan Y. Molecular Cancer.2020;19(1). CrossRef
- Pomegranate for Prevention and Treatment of Cancer: An Update Sharma P, McClees SF , Afaq F. Molecules (Basel, Switzerland).2017;22(1). CrossRef
- Cannabis and its constituents for cancer: History, biogenesis, chemistry and pharmacological activities Lal S, Shekher A, Puneet n, Narula AS , Abrahamse H, Gupta SC . Pharmacological Research.2021;163. CrossRef
- Phytochemical-Mediated Modulation of Signaling Pathways: A Promising Avenue for Drug Discovery Paul JK , Azmal M, Shah Newaz Been Haque ANM , Talukder OF , Meem M, Ghosh A. ResearchGate.2024. CrossRef
- Luteolin inhibits proliferation, triggers apoptosis and modulates Akt/mTOR and MAP kinase pathways in HeLa cells Raina R, Pramodh S, Rais N, Haque S, Shafarin J, Bajbouj K, Hamad M, Hussain A. Oncology Letters.2021;21(3). CrossRef
- Chapter 5. Role of Phytochemicals in MAPK Signaling Pathway-Mediated Apoptosis | Request PDF Kaur S, Manish Kumar , Satwinderjeet Kaur . ResearchGate.2024. CrossRef
- Epidermal growth factor receptor: Role in human cancer Rajaram P, Chandra P, Ticku S, Pallavi B. K., Rudresh K. B., Mansabdar P. Indian Journal of Dental Research: Official Publication of Indian Society for Dental Research.2017;28(6). CrossRef
- Epidermal growth factor receptor in tumor angiogenesis Ellis LM . Hematology/Oncology Clinics of North America.2004;18(5). CrossRef
- Phytochemicals targeting epidermal growth factor receptor (EGFR) for the prevention and treatment of HNSCC: A review Li S, Sun Y. Medicine.2023;102(40). CrossRef
- Phytochemicals Targeting VEGF and VEGF-Related Multifactors as Anticancer Therapy Parveen A, Subedi L, Kim HW , Khan Z, Zahra Z, Farooqi MQ , Kim SY . Journal of Clinical Medicine.2019;8(3). CrossRef
- Plant Secondary Metabolites as Anticancer Agents: Successes in Clinical Trials and Therapeutic Application Seca AML , Pinto DCGA . International Journal of Molecular Sciences.2018;19(1). CrossRef
- Plants as sources of natural and recombinant anti-cancer agents Buyel J. F.. Biotechnology Advances.2018;36(2). CrossRef
- Plant derived secondary metabolites as anti-cancer agents Rawat DS , Singh R. Anti-Cancer Agents in Medicinal Chemistry.2013;13(10). CrossRef
- Pharmacological activity of alkaloids: a review Bribi N. Asian J Botany.2018;1(1):1-6.
- Alkaloids from Traditional Chinese Medicine against hepatocellular carcinoma Liu C, Yang S, Wang K, Bao X, Liu Y, Zhou S, Liu H, et al . Biomedicine & Pharmacotherapy = Biomedecine & Pharmacotherapie.2019;120. CrossRef
- Bisbenzylisoquinoline Alkaloids Weber C, Opatz T. The Alkaloids. Chemistry and Biology.2019;81. CrossRef
- Cinchona Alkaloids-Derivatives and Applications Boratyński PJ , Zielińska-Błajet M, Skarżewski J. The Alkaloids. Chemistry and Biology.2019;82. CrossRef
- Simultaneous inhibition of cell-cycle, proliferation, survival, metastatic pathways and induction of apoptosis in breast cancer cells by a phytochemical super-cocktail: genes that underpin its mode of action Ouhtit A, Gaur RL , Abdraboh M, Ireland SK , Rao PN , Raj SG , Al-Riyami H, et al . Journal of Cancer.2013;4(9). CrossRef
- Indole-3-carbinol (I3C) induced cell growth inhibition, G1 cell cycle arrest and apoptosis in prostate cancer cells Chinni S. R., Li Y., Upadhyay S., Koppolu P. K., Sarkar F. H.. Oncogene.2001;20(23). CrossRef
- Indole-3-carbinol enhances ultraviolet B-induced apoptosis by sensitizing human melanoma cells Kim D.-S., Jeong Y.-M., Moon S.-I., Kim S.-Y., Kwon S.-B., Park E.-S., Youn S.-W., Park K.-C.. Cellular and molecular life sciences: CMLS.2006;63(22). CrossRef
- Targets for indole-3-carbinol in cancer prevention Kim YS , Milner J. A.. The Journal of Nutritional Biochemistry.2005;16(2). CrossRef
- Molecular targets and anticancer potential of indole-3-carbinol and its derivatives Aggarwal BB , Ichikawa H. Cell Cycle (Georgetown, Tex.).2005;4(9). CrossRef
- The Cannabis Terpenes Sommano SR , Chittasupho C, Ruksiriwanich W, Jantrawut P. Molecules (Basel, Switzerland).2020;25(24). CrossRef
- Diterpenes and Their Derivatives as Potential Anticancer Agents Islam MT . Phytotherapy research: PTR.2017;31(5). CrossRef
- Resveratrol as an anti-cancer agent: A review Rauf A, Imran M, Butt MS , Nadeem M, Peters DG , Mubarak MS . Critical Reviews in Food Science and Nutrition.2018;58(9). CrossRef
- Flavonoids: an overview Panche A. N., Diwan A. D., Chandra S. R.. Journal of Nutritional Science.2016;5. CrossRef
- Quercetin mediates the down-regulation of mutant p53 in the human breast cancer cell line MDA-MB468 Avila M. A., Velasco J. A., Cansado J., Notario V.. Cancer Research.1994;54(9).
- Phase I clinical trial of the flavonoid quercetin: pharmacokinetics and evidence for in vivo tyrosine kinase inhibition Ferry D. R., Smith A., Malkhandi J., Fyfe D. W., deTakats P. G., Anderson D., Baker J., Kerr D. J.. Clinical Cancer Research: An Official Journal of the American Association for Cancer Research.1996;2(4).
- Quercetin inhibits p21-RAS expression in human colon cancer cell lines and in primary colorectal tumors Ranelletti F. O., Maggiano N., Serra F. G., Ricci R., Larocca L. M., Lanza P., Scambia G., Fattorossi A., Capelli A., Piantelli M.. International Journal of Cancer.2000;85(3).
- Quercetin inhibits heat shock protein induction but not heat shock factor DNA-binding in human breast carcinoma cells Hansen R. K., Oesterreich S., Lemieux P., Sarge K. D., Fuqua S. A.. Biochemical and Biophysical Research Communications.1997;239(3). CrossRef
- Inhibition of growth and sensitization to cisplatin-mediated killing of ovarian cancer cells by polyphenolic chemopreventive agents Chan MM , Fong , Soprano KJ , Holmes WF , Heverling H. Journal of Cellular Physiology.2003;194(1). CrossRef
- Quercetin modulates signaling pathways and induces apoptosis in cervical cancer cells Kedhari Sundaram M, Raina R, Afroze N, Bajbouj K, Hamad M, Haque S, Hussain A. Bioscience Reports.2019;39(8). CrossRef
- Antitumor and apoptotic effects of quercetin on human melanoma cells involving JNK/P38 MAPK signaling activation Kim S, Yoo E, Woo J, Han S, Lee J, Jung S, Kim H, Jung J. European Journal of Pharmacology.2019;860. CrossRef
- Role of Flavonoids as Epigenetic Modulators in Cancer Prevention and Therapy Fatima N, Baqri SSR , Bhattacharya A, Koney NK , Husain K, Abbas A, Ansari RA . Frontiers in Genetics.2021;12. CrossRef
- Polyphenols and Human Health: The Role of Bioavailability Di Lorenzo C, Colombo F, Biella S, Stockley C, Restani P. Nutrients.2021;13(1). CrossRef
- Natural Polyphenols: Chemical Classification, Definition of Classes, Subcategories, and Structures Singla Rk , Dubey Ak , Garg A , Sharm Rk , Fiorino M , Ameen Sm , Haddad Ma , Al-Hiary M . Journal of AOAC International.2019;102(5). CrossRef
- Preliminary results indicate resveratrol affects proliferation and apoptosis of leukemia cells by regulating PTEN/PI3K/AKT pathway Meng J., Liu G.-J., Song J.-Y., Chen L., Wang A.-H., Gao X.-X., Wang Z.-J.. European Review for Medical and Pharmacological Sciences.2019;23(10). CrossRef
- Resveratrol inhibits the proliferation of human melanoma cells by inducing G1/S cell cycle arrest and apoptosis Wu Z, Liu B, E C, Liu J, Zhang Q, Liu J, Chen N, Chen R, Zhu R. Molecular Medicine Reports.2015;11(1). CrossRef
- Resveratrol inhibits proliferation, migration and invasion of multiple myeloma cells via NEAT1-mediated Wnt/β-catenin signaling pathway Geng W, Guo X, Zhang L, Ma Y, Wang L, Liu Z, Ji H, Xiong Y. Biomedicine & Pharmacotherapy = Biomedecine & Pharmacotherapie.2018;107. CrossRef
- Curcumin improves the efficacy of cisplatin by targeting cancer stem-like cells through p21 and cyclin D1-mediated tumour cell inhibition in non-small cell lung cancer cell lines Baharuddin P, Satar N, Fakiruddin KS , Zakaria N, Lim MN , Yusoff NM , Zakaria Z, Yahaya BH . Oncology Reports.2016;35(1). CrossRef
- Curcumin regulates proliferation, autophagy, and apoptosis in gastric cancer cells by affecting PI3K and P53 signaling Fu H, Wang C, Yang D, Wei Z, Xu J, Hu Z, Zhang Y, Wang W, Yan R, Cai Q. Journal of Cellular Physiology.2018;233(6). CrossRef
- Curcumin Induces Apoptotic Cell Death via Inhibition of PI3-Kinase/AKT Pathway in B-Precursor Acute Lymphoblastic Leukemia Kuttikrishnan S, Siveen KS , Prabhu KS , Khan AQ , Ahmed EI , Akhtar S, Ali TA , et al . Frontiers in Oncology.2019;9. CrossRef
- Natural Forms of Vitamin E as Effective Agents for Cancer Prevention and Therapy Jiang Q. Advances in Nutrition (Bethesda, Md.).2017;8(6). CrossRef
- Vitamin E and its anticancer effects Abraham A, Kattoor AJ , Saldeen T, Mehta JL . Critical Reviews in Food Science and Nutrition.2019;59(17). CrossRef
- Multi-targeted therapy of cancer by genistein Banerjee S, Li Y, Wang Z, Sarkar FH . Cancer Letters.2008;269(2). CrossRef
- Tobacco carcinogen induces both lung cancer and non-alcoholic steatohepatitis and hepatocellular carcinomas in ferrets which can be attenuated by lycopene supplementation Aizawa K, Liu C, Tang S, Veeramachaneni S, Hu K, Smith DE , Wang X. International Journal of Cancer.2016;139(5). CrossRef
- Sulforaphane reduces YAP/∆Np63α signaling to reduce cancer stem cell survival and tumor formation Fisher ML , Ciavattone N, Grun D, Adhikary G, Eckert RL . Oncotarget.2017;8(43). CrossRef
- Dietary phytochemicals in cancer prevention and therapy: a complementary approach with promising perspectives González-Vallinas M, González-Castejón M, Rodríguez-Casado A, Ramírez de Molina A. Nutrition Reviews.2013;71(9). CrossRef
- Lycopene reduces ovarian tumor growth and intraperitoneal metastatic load Holzapfel NP , Shokoohmand A, Wagner F, Landgraf M, Champ S, Holzapfel BM , Clements JA , Hutmacher DW , Loessner D. American Journal of Cancer Research.2017;7(6).
- Serum carotenoids and colorectal cancer risk: A case-control study in Guangdong, China Huang J, Lu M, Fang Y, Xu M, Huang W, Pan Z, Chen Y, Zhang C. Molecular Nutrition & Food Research.2017;61(10). CrossRef
- Tomato lycopene and lung cancer prevention: from experimental to human studies Palozza P, Simone RE , Catalano A, Mele MC . Cancers.2011;3(2). CrossRef
- Matrine promotes liver cancer cell apoptosis by inhibiting mitophagy and PINK1/Parkin pathways Wei R, Cao J, Yao S. Cell Stress Chaperones.2018;23(6):1295-1309.
- Targeting cell cycle by β-carboline alkaloids in vitro: Novel therapeutic prospects for the treatment of cancer Ahmad I, Fakhri S, Khan H, Jeandet P, Aschner M, Yu Z. Chemico-Biological Interactions.2020;330. CrossRef
- Berberine in combination with cisplatin induces necroptosis and apoptosis in ovarian cancer cells Liu L, Fan J, Ai G, Liu J, Luo N, Li C, Cheng Z. Biological Research.2019;52(1). CrossRef
- Flavonoids as Anticancer Agents Kopustinskiene DM , Jakstas V, Savickas A, Bernatoniene J. Nutrients.2020;12(2). CrossRef
- Baicalin induces cellular senescence in human colon cancer cells via upregulation of DEPP and the activation of Ras/Raf/MEK/ERK signaling Wang Z, Ma L, Su M, Zhou Y, Mao K, Li C, Peng G, et al . Cell Death & Disease.2018;9(2). CrossRef
- Apoptosis-Inducing Activity and Antiproliferative Effect of Gossypin on PC-3 Prostate Cancer Cells Cinar I. Anti-Cancer Agents in Medicinal Chemistry.2021;21(4). CrossRef
- Ginsenoside Rg3 Inhibition of Thyroid Cancer Metastasis Is Associated with Alternation of Actin Skeleton Wu W, Zhou Q, Zhao W, Gong Y, Su A, Liu F, Liu Y, Li Z, Zhu J. Journal of Medicinal Food.2018;21(9). CrossRef
- Cucurbitacin B suppresses proliferation of pancreatic cancer cells by ceRNA: Effect of miR-146b-5p and lncRNA-AFAP1-AS1 Zhou J, Liu M, Chen Y, Xu S, Guo Y, Zhao L. Journal of Cellular Physiology.2019;234(4). CrossRef
- Uncovering the anti-NSCLC effects and mechanisms of gypenosides by metabolomics and network pharmacology analysis Qi Y, Xie J, Xie P, Duan Y, Ling Y, Gu Y, Piao X. Journal of Ethnopharmacology.2021;281. CrossRef
- Plumbagin, a plant derived natural agent inhibits the growth of pancreatic cancer cells in in vitro and in vivo via targeting EGFR, Stat3 and NF-κB signaling pathways Hafeez BB , Jamal MS , Fischer JW , Mustafa A, Verma AK . International Journal of Cancer.2012;131(9). CrossRef
- Dihydroartemisinin is cytotoxic to papillomavirus-expressing epithelial cells in vitro and in vivo Disbrow GL , Baege AC , Kierpiec KA , Yuan H, Centeno JA , Thibodeaux CA , Hartmann D, Schlegel R R. Cancer Research.2005;65(23). CrossRef
- Dihydroartemisinin inhibits angiogenesis induced by multiple myeloma RPMI8226 cells under hypoxic conditions via downregulation of vascular endothelial growth factor expression and suppression of vascular endothelial growth factor secretion Wu X, Zhou H, Lee J. Anti-Cancer Drugs.2006;17(7). CrossRef
- Dihydroartemisinin exerts cytotoxic effects and inhibits hypoxia inducible factor-1alpha activation in C6 glioma cells Huang X, Ma Z, Zhang W, Lu Y, Wei E. The Journal of Pharmacy and Pharmacology.2007;59(6). CrossRef
- Status of novel drug delivery technology for phytotherapeutics Musthaba SM , Baboota S, Ahmed S, Ahuja A, Ali J. Expert Opinion on Drug Delivery.2009;6(6). CrossRef
- PGMD/curcumin nanoparticles for the treatment of breast cancer Kumari M, Sharma N, Manchanda R, Gupta N, Syed A, Bahkali AH , Nimesh S. Scientific Reports.2021;11(1). CrossRef
- Preparation and characterization of (-)-Epigallocatechin-3-gallate (EGCG)-loaded nanoparticles and their inhibitory effects on Human breast cancer MCF-7 cells Zeng L, Yan J, Luo L, Ma M, Zhu H. Scientific Reports.2017;7. CrossRef
- Bovine Serum Albumin Nanoparticles for the Efficient Delivery of Berberine: Preparation, Characterization and In vitro biological studies | Request PDF Solanki R, Patel K, Patel SJC . ResearchGate.2024. CrossRef
- Autophagy and multidrug resistance in cancer Li Y, Lei Y, Yao N, Wang C, Hu N, Ye W, Zhang D, Chen Z. Chinese Journal of Cancer.2017;36(1). CrossRef
- The multi-factorial nature of clinical multidrug resistance in cancer Assaraf YG , Brozovic A, Gonçalves AC , Jurkovicova D, Linē A, Machuqueiro M, Saponara S, Sarmento-Ribeiro AB , Xavier CPR , Vasconcelos MH . Drug Resistance Updates: Reviews and Commentaries in Antimicrobial and Anticancer Chemotherapy.2019;46. CrossRef
- Mechanisms of Multidrug Resistance in Cancer Chemotherapy Bukowski K, Kciuk M, Kontek R. International Journal of Molecular Sciences.2020;21(9). CrossRef
- Phospholipids and cholesterol: Inducers of cancer multidrug resistance and therapeutic targets Kopecka J, Trouillas P, Gašparović AC , Gazzano E, Assaraf YG , Riganti C. Drug Resistance Updates: Reviews and Commentaries in Antimicrobial and Anticancer Chemotherapy.2020;49. CrossRef
- ABC Transporter-Mediated Multidrug-Resistant Cancer Amawi H, Sim H, Tiwari AK , Ambudkar SV , Shukla S. Advances in Experimental Medicine and Biology.2019;1141. CrossRef
- Moxifloxacin-isatin Hybrids Tethered by 1,2,3-triazole and their Anticancer Activities Yang M, Liu H, Zhang Y, Wang X, Xu Z. Current Topics in Medicinal Chemistry.2020;20(16). CrossRef
- Pyrimidine: A promising scaffold for optimization to develop the inhibitors of ABC transporters He Z, Zhao T, Gong Y, Zhang X, Ma L, Liu H. European Journal of Medicinal Chemistry.2020;200. CrossRef
- Natural products as multidrug resistance modulators in cancer Kumar A, Jaitak V. European Journal of Medicinal Chemistry.2019;176. CrossRef
- LncRNA as a multifunctional regulator in cancer multi-drug resistance He J, Zhu S, Liang X, Zhang Q, Luo X, Liu C, Song L. Molecular Biology Reports.2021;48(8). CrossRef
- The potential of multi-compound nanoparticles to bypass drug resistance in cancer Da Silva C. G., Peters GJ , Ossendorp F, Cruz LJ . Cancer Chemotherapy and Pharmacology.2017;80(5). CrossRef
- Mechanisms of Chemotherapy-Induced Peripheral Neuropathy Zajączkowska R, Kocot-Kępska M, Leppert W, Wrzosek A, Mika J, Wordliczek J. International Journal of Molecular Sciences.2019;20(6). CrossRef
- Synthesis and biological evaluation of quercetin-zinc (II) complex for anti-cancer and anti-metastasis of human bladder cancer cells Lee Y, Tuyet P. In Vitro Cellular & Developmental Biology. Animal.2019;55(6). CrossRef
- Irinotecan: 25 years of cancer treatment Bailly C. Pharmacological Research.2019;148. CrossRef
- In vitro and in vivo antitumoral effects of combinations of polyphenols, or polyphenols and anticancer drugs: perspectives on cancer treatment Fantini M, Benvenuto M, Masuelli L, Frajese GV , Tresoldi I, Modesti A, Bei R. International Journal of Molecular Sciences.2015;16(5). CrossRef
- Carrier-Enhanced Anticancer Efficacy of Sunitinib-Loaded Green Tea-Based Micellar Nanocomplex beyond Tumor-Targeted Delivery Yongvongsoontorn N, Chung JE , Gao SJ Shu Jun, Bae KH , Yamashita A, Tan M, Ying JY , Kurisawa M. ACS nano.2019;13(7). CrossRef
License

This work is licensed under a Creative Commons Attribution-NonCommercial 4.0 International License.
Copyright
© Asian Pacific Journal of Cancer Biology , 2025
Author Details