Nanoliposomes Meet Folic Acid: A Precision Delivery System for Bleomycin in Cancer Treatment
Download
Abstract
Overview: The targeted administration of anticancer therapies, particularly through folate receptor (FR)-mediated targeting, enhances treatment effectiveness while minimizing side effects. This study assesses the therapeutic potential of folate-targeted liposomal bleomycin (FL-BLM) against traditional forms in treating human ovarian carcinoma and oral cancer.
Methods: FL-BLM was created using the thin film hydration technique with folic acid integration for active targeting. Its efficacy was compared to non-targeted liposomal bleomycin (L-BLM) and traditional bleomycin (BLM) using the MTT assay and flow cytometry to measure G2/M phase cell cycle arrest.
Results: FL-BLM demonstrated significantly greater effectiveness in reducing cell viability and inducing G2/M phase arrest in oral cancer cells (HN cells, OECM-1) and ovarian cancer cells (A2780CP) compared to L-BLM and BLM, indicating successful folate-mediated targeting.
Conclusions: FL-BLM effectively targets and inhibits FR-overexpressing cancer cells, particularly in both cancers. This supports the potential of folate-mediated targeting in liposomal drug delivery systems for improving drug delivery and reducing toxicity. Future research should further explore FR-targeted therapies across various cancer types
Introduction
Cancer continues to be one of the foremost health challenges worldwide, causing millions of deaths each year [1]. The principal goal of cancer therapy is to maximize efficacy while minimizing the adverse effects associated with treatment [2-3]. Traditional chemotherapy, though potent, often lacks specificity, leading to significant systemic toxicity and collateral damage to healthy tissues. To overcome these limitations, targeted drug delivery systems have emerged as a pivotal innovation, promising to enhance therapeutic precision and reduce side effects [4-5]. Millions globally are affected by oral cancer, a highly aggressive and often deadly disease with limited treatment choices [6-9]. Among the various strategies for targeted therapy, folate receptor (FR)-mediated targeting stands out [10]. In many cancer types, folate receptors are often elevated in cancers of the ovary, kidney, lung, mammary gland, brain, endometrium, and myeloid cells of hematopoietic origin. This characteristic can be leveraged to direct therapies specifically to malignant cells [11-12]. Folate-conjugated molecules are particularly adept at binding to and being internalized by cells that express these receptors, thereby delivering the therapeutic agents directly to the tumor site [13]. Bleomycin (BLM) is an anticancer agent used to treat several cancers but is hampered by its lack of targeting specificity and severe side effects, such as pulmonary toxicity [14-15].
Encapsulating BLM within liposomes can mitigate these issues, and further functionalizing these liposomes with folic acid to create Folate-Targeted Liposomal Bleomycin (FL-BLM) aims to enhance drug delivery precision. This advanced formulation promises to concentrate the drug at the tumor site, thereby increasing efficacy and minimizing systemic exposure [16]. This study explores the development of FL-BLM using the thin film hydration technique, incorporating folic acid for targeted delivery. By comparing the therapeutic outcomes of FL-BLM with non-targeted liposomal bleomycin (L-BLM) and conventional bleomycin (BLM), we aim to demonstrate the benefits of folate-mediated targeting. Specifically, we assess the capability of FL-BLM to induce G2/M phase arrest a critical phase where cells can be halted before division, offering a strategic target for cancer treatment. The potential of FL-BLM to enhance targeting accuracy, improve drug efficacy, and reduce toxicity in cancer therapy is critically evaluated, highlighting its significance in the evolution of cancer treatment paradigms.
Materials and Methods
Materials
The following materials were sourced from various suppliers: DSPC from Lipoid GmbH (Germany), DSPE- mPEG2000 and DSPE-PEG (2000)-Folate from Avanti Polar Lipids Company (USA), Bleomycin from Cell Pharma GmbH (Germany), and several reagents from Sigma-Aldrich Corporation, Merck, and Invitrogen Corporation, while the cell lines OECM-1 and A2780CP were obtained from the National Cell Bank of Iran.
Preparation of nanoliposomes
Nanoliposomes, either unencapsulated or encapsulating a drug, were produced via the thin-film hydration technique. Initially, specific molar ratios of DSPC/ cholesterol/DSPE-mPEG2000, equating to weights of 100 mg, 14 mg, and 24 mg respectively, were dissolved in 14 ml of a MeOH mixture. This mixture was then positioned in a round-bottom flask and evaporated under a rotary evaporator at 60 ºC and 120 rpm. Following evaporation, the film was dried with a nitrogen gas flush and subsequently rehydrated in phosphate buffer saline (PBS, pH 7.2) either with 5 mg of drug for the test sample or without for the blank control sample, and heated at 60 °C in a water bath. To ensure focused distribution,
3.5 mg of FA-PEG2000-DSPE was incorporated into the suspension using the post-insertion technique as shown in figure 1.
Figure 1.The Diagram of Preparation of Nanoliposomes.
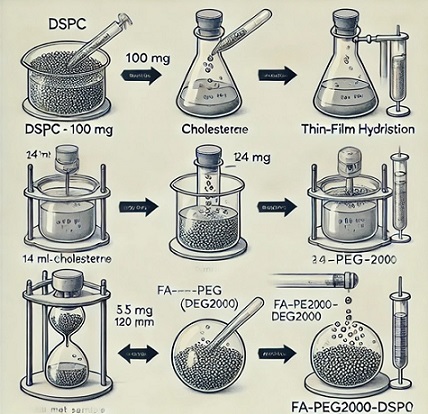
Drug-loaded nanoliposomes devoid of FA-PEG2000-DSPE, designated as L-BLM, were also fabricated as controls to assess the targeting efficiency of folate-targeted liposomal bleomycin (FL-BLM). In preparing L-BLM, the amount of mPEG2000-DSPE was raised from 24 mg to 28 mg. Finally, liposomal BLM was isolated from free BLM using a Sephadex G-50 column balanced with PBS (pH 7.2).
Characterization of nanoliposomes
To ascertain the average particle size of the created liposomal formulation loaded with anticancer drug, a dilution factor of 1:15 was applied utilizing Phosphate Buffered Saline set to pH 7.2. Post absorbance quantification at 633 nanometers wavelength, the particles’ dimensions alongside surface charge characteristics were scrutinized with the assistance of a specialized Zetasizer instrument (model NANO ZS3600; manufactured by Malvern Instruments based in the United Kingdom).
In vitro Drug Release Study
The influence of the carrier on drug release behavior was assessed through a membrane diffusion technique in phosphate buffer saline (PBS). Specifically, 2 ml of the drug formulation containing 0.8 mg of the drug was sealed in dialysis bags (cellulose membrane, 10 kD cutoff) and immersed in 65 ml of PBS at pH 7.2. The experimental setup was maintained at 37 °C with continuous stirring at 100 rpm using a magnetic stirrer. Aliquots were periodically taken from the buffer chamber and replaced with fresh PBS. Before analysis, these aliquots were filtered through a 0.45 µm syringe filter. Drug concentrations in each aliquot were measured using high-performance liquid chromatography (HPLC), and drug release profiles were presented in comparison to the controls using a standard curve.
In vitro cytotoxicity studies
The cytotoxicity of different nanoliposomal bleomycin (BLM) formulations on OEMC-1 and A2780CP cell lines was assessed through the MTT assay. Cells were cultured in a medium with 10% FBS, 100 μg/mL streptomycin, 100 U/mL penicillin, and 0.25 μg/mL amphotericin B, and incubated in a 5% CO2 humidified atmosphere at 37°C. Approximately 10,000 cells were seeded per well in a 96-well plate containing 200 μL of medium and allowed to attach for 24 hours. The medium was then replaced with either fresh medium (negative control) or medium with various concentrations of nanoliposomal BLM, followed by a 48-hour incubation. At the end of this period, the medium was replaced with 180 μL of fresh medium and 20 μL of MTT solution (5 mg/mL in PBS) for a 3-hour incubation. The reaction was stopped by adding 200 μL of DMSO to dissolve the formazan crystals. Optical density was read at 570 nm using an AccuReader microplate reader (M965 Series, Metertech, Taipei, Taiwan), and IC50 values were calculated with GraphPad Prism 6 (GraphPad Software Inc., San Diego, CA).
Cellular uptake study
The study on the vital role of cellular uptake of drug-loaded vesicles in their biological effects involved evaluating the efficiency of cell absorption. To determine this, cells were exposed to FL-BLM and L-BLM at temperatures of 37°C for two hours. OEMC-1 and A2780CP cells were initially cultured at a density of 3 × 105 cells per well in 24-well plates, either in a medium without folate or one containing 1 mM folic acid. Following an overnight incubation, the cells were promptly washed with cold PBS and a pH 3.5 saline solution (130 mM NaCl, 20 mM NaOAc) to release any folate bound to receptors.
Then, 1 mL of fresh medium with 0.07 mg/mL BLM in various formulations (FL-BLM, L-BLM, and standard BLM) was added to the existing medium, and the cells were incubated again for two hours at either 37°C or 4°C. After incubation, non-adherent carriers were removed by washing the cells twice with cold PBS (pH 7.4). The cells were then collected by centrifuging for five minutes at 10,000 rpm. The cell pellet was lysed with 200 µL of 0.5% Triton X-100 after discarding the supernatant. An equal volume of acetonitrile was added to the lysate (1:1 v/v) to precipitate proteins. Following another centrifugation at 10,000 rpm for five minutes and filtration through a 0.22 µm filter, 20 µL of the filtrate was analyzed by HPLC to measure cellular uptake efficiency.
Equation 1: Cellular Uptake Eficiency (CUE %)
CUE %: the amount of BLM in the cells after incubation time /amount of BLM in the nanoliposomes inserted into the cells× 100
Cell cycle analysis
The influence of nanoliposomes on cell cycle progression was assessed through DNA flow cytometry. Roughly 5 × 105 OEMC-1 and A2780CP cells were seeded in six-well culture plates and kept at 37 °C in a humidified 5% CO2 atmosphere for 24 hours. The medium was then replaced with 2 µM of conventional BLM and FL-BLM, and cells were incubated for an additional 48 hours. Following incubation, the medium was discarded, and the cells were trypsinized, rinsed, and collected. They were fixed in 70% cold ethanol on ice for at least 2 hours, then centrifuged at 200×g for 5 minutes to form a pellet. The pellet was resuspended in PBS with 20 µg/ mL propidium iodide (PI), 1 mg/mL RNase, and 0.1% Triton X-100 and incubated at 37 °C for 15 minutes in the dark. DNA content and cell cycle phase distribution were examined using a Partec GmbH flow cytometer (Munster, Germany) with FlowJo software (FlowJo, LLC, Ashland, OR), with appropriate gating strategies employed to exclude cell debris.
Statistical Analysis
The mean ± standard deviation (SD, n = 3) was used to express the data. Using SPSS software version 19, ANOVA and Student’s t-test were used for statistical evaluations, with a significance threshold set at p < 0.05.
Results
Characteristics of Nanoparticles
The physicochemical characteristics of nanoliposomal BLM are presented in Table 1, which includes parameters like polydispersity index (PDI), mean particle size, and zeta potential.
Formulations | Size (nm) | Zeta Potential (mV) | PDI |
FL-BLM | 185±6.2 | -32±3.3 | 0.20±0.1 |
Each value demonstrates the mean ± SD (n=3).
Drug Release Study
The release of BLM from the FL-BLM and L-BLM formulations in phosphate-buffered saline (PBS) at pH 7.2 was assessed using the membrane diffusion method. Both formulations showed a consistent release pattern with no initial burst effect. After 42 hours of dialysis in PBS, the BLM release rates were approximately 32% for FL-BLM and 34% for L-BLM, as shown in Figure 2.
Figure 2. The Release Profiles of FL-BLM, and L-BLM in Phosphate-buffered Saline (PBS) were Analyzed Using the Dialysis Method Over 42 hours at 37 ºC. The results are presented as the mean ± standard deviation (SD) with a sample size of three (n = 3).
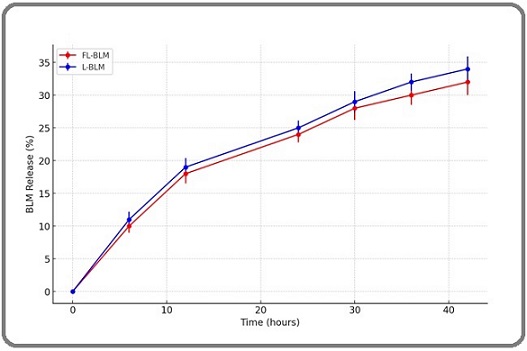
This indicates minimal differences in the release profiles of BLM between the two formulations at pH 7.2. However, the formulation released BLM at a much slower rate compared to the unencapsulated BLM formulation at both pH levels.
In vitro cytotoxicity assay
After a 48-hour incubation at 37 °C, BLM-loaded nanoliposome cytotoxicity was evaluated against conventional BLM at equivalent doses on OEMC-1 and A2780CP cells. The findings, presented in Table 2, indicated that FL-BLM exhibited notably higher cytotoxicity than conventional BLM in both A2780CP and OEMC-1 cells.
Formulations | A2780CP (IC50, µM) | OEMC-1 (IC50, µM) |
FL-BLM in folate-free medium | 22.2±1.9 | 30.7±2.9 |
FL-BLM in standard medium 1 mM folate | 48.3±2.5 | 60.8±5.5 |
FL-BLM in standard medium. | 41.2±2.3 | 58.9±5.0 |
Each value demonstrates the mean ± SD (n=3).
Cellular uptake study
The function of the folate receptor (FR) in the uptake of folate-conjugated nanoliposomes was examined through a cellular uptake investigation. As seen in Table 3, FL-BLM was significantly more readily absorbed by A2780CP and OEMC-1 cells than by L-BLM and traditional BLM.
Formulations | A2780CP (CUE %) | OEMC-1 (CUE %) |
FL-BLM | 88 | 77 |
Free-BLM | 38 | 40 |
L-BLM | 37 | 42 |
Cell Cycle Analysis
BLM demonstrates anticancer effects by inhibiting DNA synthesis, leading primarily to cell cycle arrest in the G2/M phase. To assess the impact of folate-targeted nanoliposomes on cell cycle progression, OEMC-1 and A2780CP cells were exposed to 2 μM of FL-BLM and standard BLM for 48 hours. Flow cytometry analysis indicated a statistically significant rise in the percentage of cells arrested in the G2/M phase with FL-BLM treatment compared to conventional BLM (Tables 4 and 5).
Formulations | Sub-G1% | G0/G1% | S% | G2/M% |
FL- BLM | 9.46 ± 2.08 | 19.52 ± 3.8 | 52.37 ± 5.5 | 55.32 ± 4.8 |
Conventional BLM | 19.33 ± 2.8 | 37.60 ± 4.4 | 42.29 ± 4.9 | 29.52 ± 5.5 |
Each data represents the mean ± SD (n = 3).
Formulations | Sub-G1% | G0/G1% | S% | G2/M% |
FL- BLM | 3.28 ± 2.2 | 13.4 ±3.1 | 45.72 ± 3.7 | 49.92 ± 4.4 |
Conventional BLM | 15.32 ± 1.8 | 33.08 ± 2.8 | 38.01 ± 2.6 | 25.24 ± 3.4 |
Each data represents the mean ± SD (n = 3).
Discussion
Targeted drug delivery has emerged as a critical advancement in cancer therapy, primarily aimed at enhancing therapeutic efficacy while minimizing systemic side effects. Traditional chemotherapy often suffers from a lack of specificity, which can lead to significant damage to healthy tissues. To address these challenges, innovative strategies have been developed, with liposomes, particularly folate-targeted liposomes, showing remarkable potential in improving drug delivery precision [16-20]. Liposomes are spherical vesicles composed of phospholipid bilayers capable of encapsulating both hydrophilic and hydrophobic drugs. Their inherent biocompatibility and ability to enhance drug solubility render them ideal carriers for anticancer agents. Encapsulating drugs within liposomes can significantly reduce toxicity and enhance the therapeutic index by providing controlled release at the tumor site. Furthermore, the surface of liposomes can be modified with targeting ligands, such as folic acid, to achieve selective delivery to cancer cells that overexpress folate receptors (FRs). This functionalization allows for a more targeted approach, increasing the likelihood of drug uptake by malignant cells while sparing normal tissues [18, 21-25]. Folate receptors are known to be overexpressed in various cancers, including ovarian and oral cancers. The strong affinity of folic acid for these receptors enables a more precise delivery mechanism, allowing for enhanced uptake of the drug specifically in FR-overexpressing cancer cells. This targeted approach not only improves the efficacy of the drug but also reduces the potential side effects associated with systemic chemotherapy [26-28]. Humanity has long grappled with a range of diseases, both mental and physical. Throughout history, our efforts have focused on easing these challenges. Today, new technologies like artificial intelligence are transforming this pursuit, providing powerful tools for designing and discovering innovative solutions. By integrating advancements in biology and chemistry, AI is helping to open new pathways in the search for effective treatments and therapies [29-54]. One example of drug delivery systems is exosomes, which have emerged as a novel alternative for transporting small molecules [55]. Another solution for addressing disease and its related factors is modeling [56]. In medicine, modeling is also used in brain cancer diagnosis, which has significantly addressed this major human challenge [57]. Another study, modeling can also be seen in the research conducted by Ms. Jafarian [58]. One example of utilizing modeling can be seen in another study [59]. Modeling can be applied in various industries as well [60]. One disease related to healthcare practices is oral squamous cell carcinoma [61]. Healthcare plays a fundamental role in promoting individual well- being, and new methods, such as artificial intelligence, can significantly contribute to reducing diseases by considering multiple factors [62]. In addition to various types of cancer, diabetes causes a significant number of deaths in society. Research suggests that zinc and vitamin C can have effects on this disease [63]. In special issues, interpersonal communication plays an important role in enhancing learning [64]. In another issues, nurses have played a vital role in controlling this disease worldwide [65]. One example of diseases where gender plays a role is caseous calcification of the mitral annulus (CCMA), a rare form of mitral annular calcification (MAC). CCMA results from a chronic degenerative process involving the fibrous annulus of the mitral valve. Its prevalence is highest among women, especially those over the age of 85 [66]. One disease associated with memory impairment is Alzheimer’s [67]. The study presented demonstrates that folate-targeted liposomal bleomycin (FL-BLM) significantly outperformed non-targeted liposomal bleomycin (L-BLM) and conventional bleomycin (BLM) in terms of cytotoxicity against cancer cell lines A2780CP and OECM-1. The IC50 values indicate that FL-BLM has a much lower IC50, with values of 22.2 µM for A2780CP and 30.7 µM for OECM-1 in folate-free medium, compared to higher values for conventional BLM, showcasing the enhanced therapeutic efficacy of FL-BLM. Moreover, cellular uptake studies revealed that FL-BLM demonstrated superior absorption by the cancer cells, achieving a cellular uptake efficiency of 88% for A2780CP cells and 77% for OECM-1 cells, while L-BLM showed only 37% and 42%, respectively. Flow cytometry analysis further supported these findings, as treatment with FL-BLM resulted in a significant increase in G2/M phase arrest, with 55.32% of A2780CP cells and 49.92% of OECM-1 cells arrested, compared to only 29.52% and 25.24% for conventional BLM. These results underscore the effectiveness of folate-mediated targeting in enhancing drug delivery precision and reducing toxicity associated with traditional chemotherapeutic agents.
In conclusion, cancer has been one of the most serious health threats [68]. To combat it, chemotherapy has become a widely used treatment over the past few decades [69]. The development of folate-targeted liposomal formulations represents a promising approach to enhancing the specificity and efficacy of anticancer therapies. The observed improvements in cellular uptake and cytotoxicity in recent studies highlight the potential of targeted drug delivery systems in cancer treatment. However, several challenges remain. While folate-targeted therapies aim to minimize off-target effects, the presence of folate receptors in normal tissues necessitates further refinement of specificity. Additionally, cancer cells may develop resistance mechanisms, which could undermine the effectiveness of these therapies. Ensuring formulation stability during storage and circulation in the bloodstream is also critical to achieving optimal therapeutic impact upon administration.
Future research should focus on optimizing folate-targeted formulations to enhance stability, efficacy, and specificity while addressing resistance challenges. Expanding the applicability of folate-targeted therapies across various cancer types and integrating multimodal approaches may provide synergistic effects, further improving treatment outcomes.
Acknowledgements
None.
Data Availability
The data used in this study are derived from previously published articles and are therefore not applicable.
Approved by a Scientific Body
This manuscript is not part of any student thesis or study, and therefore, approval from a scientific body is not applicable.
Ethical Issues and Approval
This study did not involve any original data collection or human subjects, and therefore, ethical approval was not required.
Consent for Publication
All authors have provided consent for publication.
Conflict of Interest
The authors declare no potential conflicts of interest.
References
- Global Cancer Statistics 2020: GLOBOCAN Estimates of Incidence and Mortality Worldwide for 36 Cancers in 185 Countries Sung H, Ferlay J, Siegel RL , Laversanne M, Soerjomataram I, Jemal A, Bray F. CA: a cancer journal for clinicians.2021;71(3). CrossRef
- Cancer prevention and treatment using combination therapy with natural compounds Sauter ER . Expert Review of Clinical Pharmacology.2020;13(3). CrossRef
- Curcumin-cisplatin chemotherapy: A novel strategy in promoting chemotherapy efficacy and reducing side effects Hussain Y, Islam L, Khan H, Filosa R, Aschner M, Javed S. Phytotherapy research: PTR.2021;35(12). CrossRef
- Controlled drug delivery vehicles for cancer treatment and their performance Senapati S, Mahanta AK , Kumar S, Maiti P. Signal Transduction and Targeted Therapy.2018;3. CrossRef
- Polymeric drug delivery systems for localized cancer chemotherapy De Souza R, Zahedi P, Allen CJ , Piquette-Miller M. Drug Delivery.2010;17(6). CrossRef
- Scaffold Application for Bone Regeneration with Stem Cells in Dentistry: Literature Review Saberian E, Jenča A, Zafari Y, Jenča A, Petrášová A, Zare-Zardini H, Jenčová J. Cells.2024;13(12). CrossRef
- Comparative Analysis of Osteoblastic Responses to Titanium and Alumina-Toughened Zirconia Implants: An In Vitro Study Saberian E, Jenča A, Seyfaddini R, Jenča A, Zare-Zardini H, Petrášová A, Jenčová J. Biomolecules.2024;14(6). CrossRef
- Enhancing Cisplatin Delivery via Liposomal Nanoparticles for Oral Cancer Treatment Ghanbarikondori P, Aliakbari RBS , Saberian E, Jenča A, Petrášová A, Jenčová J, Khayavi AA . Indian Journal of Clinical Biochemistry.2024. CrossRef
- Application of Scaffold-Based Drug Delivery in Oral Cancer Treatment: A Novel Approach Saberian E, Jenča A, Petrášová A, Zare-Zardini H, Ebrahimifar M. Pharmaceutics.2024;16(6). CrossRef
- Targeting the folate receptor: diagnostic and therapeutic approaches to personalize cancer treatments Ledermann J. A., Canevari S., Thigpen T.. Annals of Oncology: Official Journal of the European Society for Medical Oncology.2015;26(10). CrossRef
- The folate receptor as a rational therapeutic target for personalized cancer treatment Assaraf YG , Leamon CP , Reddy JA . Drug Resistance Updates: Reviews and Commentaries in Antimicrobial and Anticancer Chemotherapy.2014;17(4-6). CrossRef
- Immunotherapy of folate receptor-expressing tumors: review of recent advances and future prospects Lu Y, Low PS . Journal of Controlled Release: Official Journal of the Controlled Release Society.2003;91(1-2). CrossRef
- Folic acid-conjugated fluorescent polymer for up-regulation folate receptor expression study via targeted imaging of tumor cells Qiao J, Dong P, Mu X, Qi L, Xiao R. Biosensors & Bioelectronics.2016;78. CrossRef
- Effect of Bleomycin Administration on the Development of Pulmonary Toxicity in Patients With Metastatic Germ Cell Tumors Receiving First-Line Chemotherapy: A Meta-Analysis of Randomized Studies Necchi A, Miceli R, Oualla K, Sonpavde G, Giannatempo P, Raggi D, Nicolai N, et al . Clinical Genitourinary Cancer.2017;15(2). CrossRef
- Bleomycin in the setting of lung fibrosis induction: From biological mechanisms to counteractions Della Latta V, Cecchettini A., Del Ry S., Morales M. A.. Pharmacological Research.2015;97. CrossRef
- Folic acid conjugated nanoliposomes as promising carriers for targeted delivery of bleomycin Chiani M, Norouzian D, Shokrgozar MA , Azadmanesh K, Najmafshar A, Mehrabi MR , Akbarzadeh A. Artificial Cells, Nanomedicine, and Biotechnology.2018;46(4). CrossRef
- Tumour-selective drug delivery via folate receptor-targeted liposomes Pan X, Lee RJ . Expert Opinion on Drug Delivery.2004;1(1). CrossRef
- Folate receptor-mediated celastrol and irinotecan combination delivery using liposomes for effective chemotherapy Soe ZC , Thapa RK , Ou W, Gautam M, Nguyen HT , Jin SG , Ku SK , et al . Colloids and Surfaces. B, Biointerfaces.2018;170. CrossRef
- Folate-liposome-mediated antisense oligodeoxynucleotide targeting to cancer cells: evaluation in vitro and in vivo Leamon CP , Cooper SR , Hardee GE . Bioconjugate Chemistry.2003;14(4). CrossRef
- New folate receptor targeted nano liposomes for delivery of 5-fluorouracil to cancer cells: Strong implication for enhanced potency and safety Handali S, Moghimipour E, Kouchak M, Ramezani Z, Amini M, Angali KA , Saremy S, et al . Life Sciences.2019;227. CrossRef
- A novel 5-Fluorouracil targeted delivery to colon cancer using folic acid conjugated liposomes Handali S, Moghimipour E, Rezaei M, Ramezani Z, Kouchak M, Amini M, Angali KA , Saremy S, Dorkoosh FA . Biomedicine & Pharmacotherapy = Biomedecine & Pharmacotherapie.2018;108. CrossRef
- Folate receptor-targeted liposomal nanocomplex for effective synergistic photothermal-chemotherapy of breast cancer in vivo Nguyen VD , Min H, Kim C, Han J, Park J, Choi E. Colloids and Surfaces. B, Biointerfaces.2019;173. CrossRef
- Anti-cancer activity of doxorubicin-loaded liposomes co-modified with transferrin and folic acid Sriraman SK , Salzano G, Sarisozen C, Torchilin V. European Journal of Pharmaceutics and Biopharmaceutics: Official Journal of Arbeitsgemeinschaft Fur Pharmazeutische Verfahrenstechnik e.V.2016;105. CrossRef
- Single-ligand dual-targeting irinotecan liposomes: Control of targeting ligand display by pH-responsive PEG-shedding strategy to enhance tumor-specific therapy and attenuate toxicity Wang Z, Lin X, Chi D, Xu Z, Lin G, Liu H, Sun J, He Z, Wang Y. International Journal of Pharmaceutics.2020;587. CrossRef
- A Novel Folate-Targeted Nanoliposomal System of Doxorubicin for Cancer Targeting Lohade AA , Jain RB , Iyer K, Roy SK , Shimpi HH , Pawar Y, Rajan MGR , Menon MD . AAPS PharmSciTech.2016;17(6). CrossRef
- Targeted drug delivery via the folate receptor Sudimack J., Lee R. J.. Advanced Drug Delivery Reviews.2000;41(2). CrossRef
- Gene delivery with active targeting to ovarian cancer cells mediated by folate receptor alpha He Z, Yu Y, Zhang Y, Yan Y, Zheng Y, He J, Xie Y, et al . Journal of Biomedical Nanotechnology.2013;9(5). CrossRef
- In vitro and in vivo evaluation of folate receptor-targeted a novel magnetic drug delivery system for ovarian cancer therapy Ak G, Yilmaz H, Güneş A, Hamarat Sanlier S. Artificial Cells, Nanomedicine, and Biotechnology.2018;46(sup1). CrossRef
- Prediction of Breast Cancer Recurrence With Machine Learning. In M. Khosrow-Pour, D.B.A. (Ed.), Encyclopedia of Information Science and Technology, Sixth Edition Owrang OMM , Schwarz G, Horestani FJ . Advance online publication.2025. CrossRef
- Integrating Protein Structure Prediction and Bayesian Optimization for Peptide Design. InNeurIPS 2023 Generative AI and Biology (GenBio) Workshop 2023 Manshour N, He F, Wang D, Xu D. https://openreview.net/ forum?id=CsjGuWD7hk..
- Environmental Determinants of Oral Cancer Development: An Overview Maddahi M, Ghanbarikondori P, Amiri F, Abdi N, Jahromi AM , Pour NS , Moazzam F. ResearchGate.2024. CrossRef
- Maxillary Sinus Volume in Cleft Lip and Palate Patients with and without an Oronasal Fistula using CBCT Kiaee B, Hafezi L, Karani M, Amiri F, Jamilian A. ResearchGate.2024. CrossRef
- Ultrasound Waves Effect on the Proliferation of Fibroblast Cells: Collagen Type I Expression Hormozi Moghaddam Z, Mokhtari-Dizaji M, Nilforoshzadeh MA , Bakhshandeh M. Journal of Biomedical Physics and Engineering.2023. CrossRef
- Synergistic anticancer effects of doxorubicin and metformin combination therapy: A systematic review Jalali F, Fakhari F, Sepehr A, Zafari J, Sarajar BO , Sarihi P, Jafarzadeh E. Translational Oncology.2024;45. CrossRef
- Enhancing breast cancer diagnosis accuracy through genetic algorithm-optimized multilayer perceptron | Request PDF Talebzadeh H, Talebzadeh M, Satarpour M, Jalali F, Farhadi B, Vahdatpour MS . ResearchGate.2024. CrossRef
- The AI Diagnostician: Improving Medical Diagnosis with Artificial Intelligence. Kindle. 2024;4(1):1-219. Farrokhi M, Taheri F, Adibnia E, Mehrtabar S, Rassaf Z, Tooyserkani SH , Rajabloo Y, et al . .
- Investigating the mortality trend of gastrointestinal cancers in Babol, North Iran (2013-2021) Ebrahimi P, Karami M, Delavari S, Shojaie L, Hosseini-Berneti SH , Bayani F, Moghaddasi M, Babazade O, Nikbakht H. BMC gastroenterology.2024;24(1). CrossRef
- Batch adsorption of methyl tert-butyl ether (MTBE) from aqueous solution by combined CNT and zeolite Behzadnezhad A, Ebadi T, Taheri S, Kowsari E. ResearchGate.2024. CrossRef
- Development of an electrochemical sensor for detection of lupron as a drug for fibroids treatment and uterine myoma in pharmaceutical waste and water sources | Request PDF Movahed F, Ehymayed H, Kalavi S, Shahrtash SA , Al-Hijazi A, Daemi A, Mahmoud H, Kashanizadeh M, Alsalamy A. ResearchGate.2024. CrossRef
- Beta lactam allergy and risk of surgical site infection: A systematic review and meta-analysis Salehi SA , Hajishah H, Amini MJ , Movahed F, Ebrahimi A, Rihani FSS , Dehbozorgi M, et al . Current Problems in Surgery.2024;61(11). CrossRef
- Ethical Considerations and Equipoise in Cancer Surgery Vakili-Ojarood M, Naseri A, Shirinzadeh-Dastgiri A, Saberi A, HaghighiKian SM , Rahmani A, Farnoush N, et al . Indian Journal of Surgical Oncology.2024;15(Suppl 3). CrossRef
- A comprehensive review of etiology, pathophysiologyepidemiology, and management of hair loss and its correlation with COVID-19 Faraz Changizi , Maryam Abdolmaleki , Mina Farjam , Laya Ohadi . International Journal of Medicine.2024;12(2):41-47. CrossRef
- The Effect of Pharmacist Interventions on the Antimicrobial Prevention Pattern in Vascular and Gastrointestinal Surgeries: A Prospective Study Yazd H, Ohadi L, Abdolmaleki M, Farsi Y, Pishgahi M. ResearchGate.2024. CrossRef
- Dynamic control of contractile resistance to iPSC-derived micro-heart muscle arrays Schuftan D, Kooh YKG , Guo J, Sun Y, Aryan L, Stottlemire B, Berkland C, Genin GM , Huebsch N. Journal of Biomedical Materials Research. Part A.2024;112(4). CrossRef
- Serum level measurement of progranulin in relapsing-remitting multiple sclerosis and neuromyelitis optica patients Azadani NN , Norouzi F, Hajizadeh M, Parsa S, Khalighinejad F. American Journal of Clinical and Experimental Immunology.2019;8(3).
- Hemodynamic Changes after Continuing or Omitting Regular Angiotensin Converting Enzyme Inhibitors before Cataract Surgery: A Comparative Study Khademi S, Jouybar R, Ahmadi S, Asmarian N, Ghadimi M, Salari M, Emami S. Current Hypertension Reviews.2023;19(1). CrossRef
- Comparing the effect of sedation with dexmedetomidine and propofol on sleep quality of patients after cardiac surgery: A randomized clinical trial Azarfarin R, Ziaei Fard M, Ghadimi M, Chaibakhsh Y, Yousefi M. Journal of Cardiovascular and Thoracic Research.2024;16(3). CrossRef
- A Comprehensive Review of Electromyography in Rehabilitation: Detecting Interrupted Wrist and Hand Movements with a Robotic Arm Approach Savoji K, Soleimani M, Moshayedi AJ . ResearchGate.2024. CrossRef
- Functional Outcome and Mortality Predictors in Patients with Cerebral Ischemic Infarction After Decompressive Craniectomy: Cross-Sectional Study Rostami A, Elyassirad D, Vatanparast M, Abouei Mehrizi MA , Hasanpour M, Rezaee H, Haghir A, Keykhosravi E. World Neurosurgery.2024;182. CrossRef
- Glycoprotein IIb/IIIa inhibitors in the treatment of thromboembolic events related to endovascular treatment of cerebral aneurysms-systematic review and meta-analysis Hasanpour M, Maleki S, Rezaee H, Aminzadeh B, Abbasi Shaye Z, Keykhosravi E. The Neuroradiology Journal.2024;37(2). CrossRef
- Effects of Intraventricular Vancomycin Administration on the Prevention of Ventricular Shunt Infection Hasanpour M, Rezaee H, Samini F, Keykhosravi E. Journal of Neurological Surgery. Part A, Central European Neurosurgery.2023. CrossRef
- Examine the impact of mental planning on building mental health and cognitive beliefs of bank employees Ghahjavarestani AM . ResearchGate.2024. CrossRef
- Redictive Role of Personality Dimensions on Quality of Life and Satisfaction in Patients With Gender Identity Disorder after Gender Reassignment Surgery Ghahjavarestani AHM . The scientific heritage.2024. https://orcid. org/0000-0002-0440-0509 ;135.
- Plasma NT1 tau is associated with hypometabolism in Alzheimer’s disease continuum Ghahri Lalaklou Z, Montazeri Ghahjavarestani A, Pishkari Y, Emami D. Neurology Letters.2024;3(Special Issue (Diagnostic and Therapeutic advances in Neurodegenerative diseases)). CrossRef
- The effects of tumor-derived exosomes enriched with miRNA-211a on B16F10 cells Atashzar MR , Ataollahi MR , Asad AG , Doroudgar P, Amani D. Contemporary Oncology (Poznan, Poland).2024;28(2). CrossRef
- A Multistage Stochastic Optimization Model for Resilient Pharmaceutical Supply Chain in COVID-19 Pandemic Based on Patient Group Priority. 2024 Systems and Information Engineering Design Symposium (SIEDS) Mahdavimanshadi M, Anaraki MG , Mowlai M, Ahmadirad Z. IEEE.2024;:382-387. CrossRef
- MRI Brain Cancer Image Detection: Application of an Integrated U-Net and ResNet50 Architecture. In Finkelstein, J., Moskovitch, R., Parimbelli, E. (eds), Artificial Intelligence in Medicine. AIME 2024. Lecture Notes in Computer Science, vol 14845. Springer, Cham Benchari M, Totaro MV . 2024. CrossRef
- Investigating health risk behavior disparities in the United States with finite mixture modeling Jaferian S, Farhadian L. ResearchGate.2024. CrossRef
- Design of Microfluidic Sensing and Transport Device Asgharighajari M, Amziah N, Sulaiman N, Sodeinde SA . Journal of Biomedical Engineering and Technology.2016;3(1). CrossRef
- Development of Impedance Base Microfluidic Flow Sensor Asgharighajari M, Amziah N, Sulaiman N, Sodeinde SA. International Research Journal of Engineering and Technology (IRJET).2015;2(9):680-685. https://www.irjet. net/archives/V2/i9/IRJET-V2I9112.pdf.
- Epigenetic Regulation in Oral Squamous Cell Carcinoma Microenvironment: A Comprehensive Review Mesgari H, Esmaelian S, Nasiri K, Ghasemzadeh S, Doroudgar P, Payandeh Z. Cancers.2023;15(23). CrossRef
- Examination of AI’s role in Diagnosis, Treatment, and Patient care. In Gupta, M., Kumar, R., & Lu, Z. (Eds.), Transforming Gender-Based Healthcare with AI and Machine Learning Sajjadi Mohammadabadi SM , Seyedkhamoushi F, Mostafavi M, Borhani Peikani M. CRC Press.2024;:221-238. CrossRef
- Co-administration of "L-Lysine, Vitamin C, and Zinc" increased the antioxidant activity, decreased insulin resistance, and improved lipid profile in streptozotocin-induced diabetic rats Yousefian M, Abedimanesh S, Yadegar A, Nakhjavani M, Bathaie SZ . Biomedicine & Pharmacotherapy = Biomedecine & Pharmacotherapie.2024;174. CrossRef
- Evaluating the effect of cochlear implantation age on pragmatic abilities before and after age of 3 Nikrah P, Ghareh Chahie R, Ghazvini A, Hajizadeh A. Applied Neuropsychology. Child.2024. CrossRef
- A qualitative approach to the ethical challenges of Iranian nurses during the COVID-19 pandemic Rajabipoor Meybodi A, Mohammadi M, Arjmandi H. Journal of Preventive and Complementary Medicine.2022;1(3). CrossRef
- Caseous calcification of the mitral annulus presenting with symptomatic complete heart block Nikoo MH , Danesh S, Abtahi F. Journal of Clinical Images and Medical Case Reports.2022;3(3):1743. CrossRef
- A Review on the Comparison of Working Memory Performance, Cognitive Function, and Behavioral, and Psychological Symptoms across Normal Aging, Mild Cognitive Impairment, and Alzheimer's Disease Ghayedi Z, Banihashemian K, Shirdel S, Adineh Salarvand R, Zare M, Zeinali S, Ghahri Lalaklou Z. ResearchGate.2024. CrossRef
- An experimental and theoretical approach of a novel phosphoramide containing naphthoquinone with potential cytotoxic and apoptosis-inducing ability Gholivand K, Faraghi M, Vahabirad M, Malekshah RE , Narimani S, Roohzadeh R, Fallah N, Jannesar S, Yousefian M. Journal of Molecular Structure.2025;1319. CrossRef
- Experimental and theoretical evaluation of biological properties of a phosphoramide functionalized graphene oxide | Request PDF Gholivand K, Barzegari A, Yousefian M, Malekshah RE , Faraghi M. ResearchGate.2024. CrossRef
License

This work is licensed under a Creative Commons Attribution-NonCommercial 4.0 International License.
Copyright
© Asian Pacific Journal of Cancer Biology , 2024
Author Details