Enhancing the Cytotoxic Effects of Carboplatin and Cisplatin on Liposomes in Oral Cancer Cells with Curcumin
Download
Abstract
Background: Oral squamous cell carcinoma remains challenging to treat effectively with conventional chemotherapy, leading researchers to explore synergistic combinations to enhance therapeutic outcomes. Combining curcumin with platinum-based drugs, such as cisplatin and carboplatin, has demonstrated potential in enhancing cytotoxic effects. However, limited studies have explored these combinations’ efficacy and sustained release profile in liposomal form specifically for oral cancer. This study investigates the enhanced cytotoxic effects of cisplatin-curcumin and carboplatin-curcumin nanoliposome formulations on CAL 27 oral cancer cells.
Methods and Materials: Nanoliposomes encapsulating cisplatin or carboplatin with curcumin were formulated and characterized by particle size, zeta potential, and polydispersity index (PDI) to ensure optimized delivery properties. Particle sizes of the cisplatin and carboplatin nanoliposomes ranged from 175 to 187 nm, with a zeta potential greater than -30 mV, indicating good stability, and PDI values less than 0.48, suggesting uniform particle size distribution. In vitro cytotoxicity was assessed using the MTT assay at 24, 48, and 96 hours across different curcumin concentrations.
Results: Cisplatin-curcumin and carboplatin-curcumin nanoliposome formulations demonstrated significantly increased cytotoxicity in CAL 27 cells compared to control groups. Drug release studies indicated a sustained release profile, with approximately 22% of cisplatin and 28% of carboplatin released over 52 hours, which may prolong therapeutic effects by maintaining drug availability within the cancer cells.
Conclusion: The findings suggest that cisplatin-curcumin and carboplatin-curcumin nanoliposomal formulations enhance the cytotoxic effects of these chemotherapeutic agents while providing a stable, sustained release profile.
Introduction
Oral cancer, especially oral squamous cell carcinoma (OSCC), is one of the most prevalent cancers worldwide, with high morbidity and mortality rates [1]. Despite advancements in treatments like surgery, radiation, and chemotherapy, the prognosis for patients with advanced oral cancer remains poor, with frequent recurrences and notable side effects from standard therapies [2-3]. Developing more efficient and less toxic treatments is essential for enhancing patient outcomes. Chemotherapy drugs, such as cisplatin and carboplatin, are commonly used in oral cancer therapy due to their ability to cause DNA damage and trigger cancer cell death. However, their effectiveness is frequently restricted by systemic toxicity and undesirable side effects, including kidney damage, hearing loss, and bone marrow suppression [4-7]. To overcome these challenges, novel drug delivery systems such as liposomal formulations have gained considerable interest in recent years. Liposomes, spherical vesicles composed of a lipid bilayer, can encapsulate both hydrophilic and hydrophobic drugs, improving their solubility, stability, and bioavailability [8-9]. Liposomal drug delivery systems can potentially enhance the therapeutic index of chemotherapy drugs by boosting drug concentration in tumor tissues via the enhanced permeability and retention (EPR) effect, while minimizing systemic toxicity [10-11]. Significantly, liposomal formulations of cisplatin and carboplatin have demonstrated promising outcomes in preclinical studies for treating various cancers, including oral cancer [12]. Curcumin, a natural polyphenol derived from the rhizome of Curcuma longa (turmeric), has gained significant attention for its wide range of pharmacological benefits, such as anti-inflammatory, antioxidant, and anticancer activities [13]. It demonstrates its anticancer properties through multiple mechanisms, including the inhibition of cancer cell growth, the induction of apoptosis, and the suppression of angiogenesis and metastasis [14]. Interestingly, curcumin has been found to boost the cytotoxic effects of chemotherapy drugs like cisplatin and carboplatin in various cancer cell lines by modulating critical signaling pathways, including NF-κB, PI3K/ Akt, and MAPK [15-16]. Nevertheless, its clinical application has been limited due to poor bioavailability and fast metabolism [17]. Recent research has focused on integrating curcumin into liposomal formulations of chemotherapy drugs to overcome these challenges. Encapsulating curcumin in liposomes can enhance its bioavailability, improve stability, and allow for controlled release, ultimately boosting its therapeutic effectiveness [18]. Moreover, the combination of curcumin with liposomal formulations of cisplatin and carboplatin holds the potential to synergistically enhance the cytotoxic impact on cancer cells, providing a more effective treatment approach with reduced side effects [19-20]. In oral cancer treatment, the combination of platinum drugs with curcumin-loaded liposomes presents a promising strategy to enhance chemotherapy’s cytotoxicity while minimizing harm to healthy tissues. Studies suggest that curcumin can increase oral cancer cells’ sensitivity to platinum-based drugs by reducing the expression of drug resistance proteins and promoting the intracellular accumulation of these platinum compounds [21-22]. Additionally, curcumin has been demonstrated to inhibit the growth of HSC-3 oral cancer cells, a recognized OSCC cell line, and induce apoptosis by activating caspases and modulating the balance between pro-apoptotic and anti-apoptotic proteins [23]. Liposomal formulations of anticancer drugs have demonstrated more efficient delivery to oral cancer cells, leading to enhanced cytotoxic effects and reduced systemic toxicity compared to free drug formulations [24-25]. When combined with curcumin, these liposomal formulations can boost antitumor activity by targeting multiple cancer pathways simultaneously, increasing drug retention within the tumor, and reducing chemotherapy resistance. This combination therapy holds significant promise for cancer treatment and warrants further investigation in preclinical and clinical studies [21, 26-29]. Recent studies indicate that curcumin-loaded liposomes can effectively enhance the cytotoxicity of chemotherapeutic agents in cancer cells. For example, one study demonstrated that curcumin- loaded nanoparticles (Cur-NPs) induce apoptosis in cisplatin-resistant oral cancer cells by suppressing MDR1 protein expression and increasing reactive oxygen species, leading to enhanced cytotoxicity and cell death [22]. Another study used liposomal co-encapsulation of curcumin with paclitaxel, showing that curcumin enhanced the efficacy of paclitaxel by targeting NF-κB pathways, which resulted in increased cytotoxicity against cancer cells [30]. Additionally, research with curcumin-loaded lipid nanocapsules revealed a significant increase in cytotoxicity in glioma cells compared to free curcumin, indicating enhanced bioavailability and therapeutic potential through encapsulation [31]. Moreover, the use of liposomal curcumin with cisplatin also showed increased apoptosis and cell death in breast cancer models, suggesting potential efficacy in similar tumor environments [32].
Materials and Methods
Experimental design
This study evaluates the cytotoxic effects of nanoliposomal formulations containing cisplatin- curcumin and carboplatin-curcumin on CAL 27 oral cancer cells. To provide a comprehensive assessment, control experiments will include non-encapsulated cisplatin, carboplatin, and curcumin alone, enabling a comparative analysis of the relative efficacy of liposomal formulations. Key parameters, including particle size, zeta potential, polydispersity index (PDI), and drug release profiles, will be assessed to gauge the potential of these formulations in enhancing the cytotoxicity of chemotherapeutic agents. The findings aim to contribute valuable insights into the use of liposomal drug delivery systems in combination with curcumin for more effective and targeted oral cancer therapies.
Carboplatin and cisplatin were sourced from Kunming Precious Metal Institute (Kunming, Yunnan, China). Cholesterol and polyethylene glycol 3350 were obtained from Sigma-Aldrich Co. (UK) and Kimyagaran Emrooz Chemical Ind. Co. (Iran), respectively. Lecithin was purchased from Acros Co. (Belgium), while RPMI 1640 cell culture medium came from Gibco Co. (Germany). The 3-(4,5-dimethylthiazol-2-yl)-2,5-diphenyl tetrazolium bromide (MTT) was supplied by Sigma-Aldrich (USA). The CAL 27 cell line was provided by the Pasteur Institute of Iran’s cell bank. All materials used were of analytical grade, and distilled water was utilized throughout the study.
Nanoliposomal Drug Preparation
Liposomal nanoparticles were prepared using the reverse phase evaporation method. In brief, lecithin, cholesterol, PEG 3350, and carboplatin were dissolved in a 1:2 methanol: ethanol mixture at molar ratios of 14, 8, 2, and 2, respectively. The solvent was then removed under vacuum using a rotary evaporator (120 rpm, 45˚C), forming a thin film in the round-bottom flask. Phosphate- buffered saline (PBS) was added to the film to form drug- loaded liposomal nanoparticles. The final concentrations of lecithin, cholesterol, PEG 3350, and carboplatin were 17, 12, 2.2, and 2.1 mM, respectively. The nanoparticle suspension was then sonicated for five minutes using a bath sonicator (50 W, Bandelin Sonopuls HD 2070, Bandelin Elec., Germany). The nanoliposome formulation of cisplatin was prepared following the same method, substituting cisplatin for carboplatin. Blank nanoparticles were prepared using the same method, except the drug was omitted.
Nanoparticle Characterization
The liposomal formulations’particle size, zeta potential, and polydispersity index (PDI) were determined at room temperature using a Zeta PALS analyzer (Brookhaven Instruments Corporation, Austin, TX), with each parameter measured three times. The levels of cisplatin and carboplatin in these formulations were quantified using an atomic absorption spectrometer and an F-2500 fluorescence spectrophotometer (Hitachi, Japan), respectively. For assessing encapsulation efficiency, the formulations underwent several centrifugation cycles at 4°C and 14,000 RPM for 30 minutes, and the supernatant was discarded after each cycle. The encapsulation efficiency was then calculated by subtracting the amount of free cisplatin and carboplatin from the total drug content, using the formula:
EE% = [(Total Anti-Cancer Drugs - Free Anti-Cancer Drugs) / Total Anti-Cancer Drugs] × 100.
In vitro Drug Release Study
The effect of the carrier on drug release was evaluated in a drug discharge study using the membrane diffusion method in phosphate-buffered saline (PBS). For this experiment, 2 ml of each drug formulation, containing 0.10 mg of the drug, along with control samples and the free drug, were sealed in dialysis bags (cellulose membrane, 12 kDa cutoff) and placed in 120 ml of PBS at pH 7.2 and pH 5.2. The setup was maintained at a temperature of 37 °C and stirred continuously at 120 rpm using a magnetic stirrer. At specific time points, aliquots were taken from the buffer chamber and replaced with fresh PBS. Before analysis, these samples were filtered through a 0.45 µm syringe filter. The drug concentration in each aliquot was determined by high-performance liquid chromatography (HPLC), and the drug release profiles were compared to controls using a standard curve.
Investigation of cytotoxicity
In this study, the CAL 27 cell line was cultured in a humidified atmosphere with 5% CO₂, using RPMI-1640 medium supplemented with 10% FBS and penicillin/ streptomycin (0.1 and 0.06 mg/ml, respectively) at a density of 1 × 10⁴ cells per well in 96-well plates. After 24 hours, the culture medium was replaced with a mixture of stock solutions containing varying concentrations of curcumin (2, 4, and 8 mM, 98% purity) in DMSO, along with fixed amounts of cisplatin and carboplatin (16 µM each) and nano-cisplatin and nano-carboplatin (16 µM each) with curcumin (2, 4, and 8 mM). After 24, 48, and 72 hours, the mixture was removed, and 20 µl of MTT solution (0.5 mg/ml in PBS) was added to each well for a 3-hour incubation. Formazan crystals were then dissolved in 100% isopropanol for 10 minutes, and absorbance was measured at 540 nm using an ELISA reader (Organon Teknika, Netherlands). Cell viability was calculated using a specified formula, and the IC50 value was determined with the Pharm program.
Statistical Analysis
The results were processed using SPSS software version 18. Data are presented as Mean ± SD from three separate experiments. Group comparisons were performed using the t-test, with the significance threshold set at 0.05.
Results
Nanoparticles characterization
The particle size of the Cisplatin nanoliposome was 175.4±14.4 nm, with a zeta potential of -30±2.7 mV and a PDI of 0.33±0.4. The Carboplatin nanoliposome had a particle size of 187.7 ±11.1 nm, a zeta potential of -32.2± 3.7 mV, and a PDI of 0.48±0.9. Additionally, the encapsulation efficiency of cisplatin and carboplatin was estimated to be 84.5 ± 3.5% and 76.5 ± 1.4%, respectively, indicating that approximately 10% more cisplatin was encapsulated in the nanoliposomes than carboplatin.
Drug release study
The release of cisplatin and carboplatin from the nanoparticle formulations in phosphate-buffered saline (PBS) at pH 7.2 and pH 5.2 was assessed using the membrane diffusion method. Both formulations exhibited a consistent release pattern, without any initial burst. After 52 hours of dialysis in PBS at different pH levels, the release of cisplatin and carboplatin from the liposome was about 22% and 28 % at pH 7.2, while the release of cisplatin and carboplatin from the liposome was around 44% and 57% at pH 5.2 (Figure 1).
Figure 1. Cumulative Release Profile of Cisplatin and Carboplatin from Nanoliposomes in Different pH Environments. The findings are reported as mean ± standard deviation (SD) with a sample size of three (n = 3).
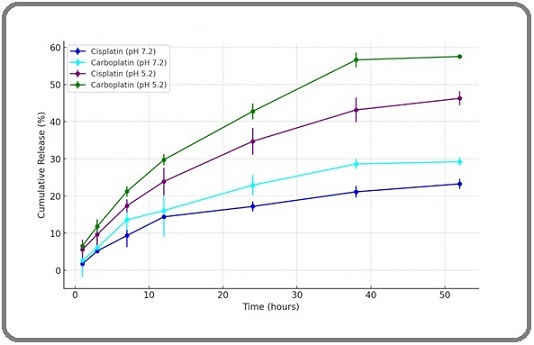
This data highlights a significant variation in the release behavior of cisplatin and carboplatin from nanoliposomes at pH 5.2, while the differences at pH 7.2 were minimal.
Investigation of cytotoxicity
Analyzing Figure. 2 which shows cell viability for various treatments over 24, 48, and 72 hours provides several key insights into the cytotoxic effects of curcumin, cisplatin, and carboplatin, both alone (control experiments) and in combination (liposomal formulations).
Figure 2. Cell Viability was Assessed by MTT Assay in Various Incubation Times at 24, 48 and 72h.The findings are reported as mean ± standard deviation (SD) with a sample size of three (n = 3).
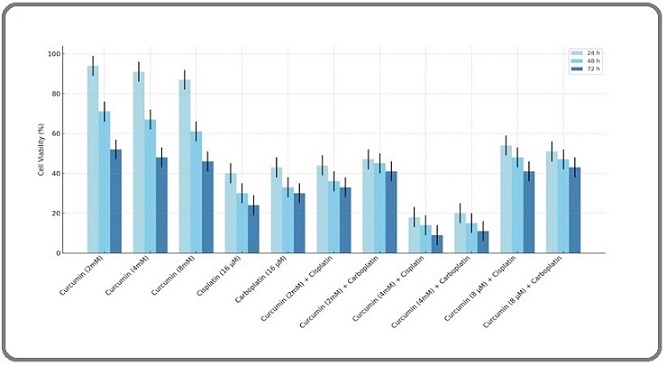
Firstly, the data indicates a clear dose-response relationship, where higher doses of curcumin consistently reduce cell viability more effectively across all time points, illustrating its dose-dependent cytotoxic nature. The platinum-based drugs, cisplatin and carboplatin, also show significant cytotoxicity at a single concentration (16 µM), with cisplatin appearing slightly more potent. Moreover, the combinations of curcumin with nano-cisplatin or nano-carboplatin enhance the cytotoxic effects beyond those observed with any single agent, suggesting potential synergistic interactions that could be leveraged for therapeutic benefit. This is particularly notable in treatments such as Curcumin (4 mM) combined with nano-cisplatin and nano-carboplatin, which significantly lowers cell viability compared to either agent alone. The comparison of the 8 mM curcumin combination with nano-carboplatin and nano-cisplatin demonstrated antagonistic effects (P<0.05). The chart also highlights the time-dependent effects of these treatments, as reductions in cell viability become more pronounced over time. This progression is critical for understanding the sustained impact of these compounds on cell survival. Additionally, the presence of error bars indicates variability in the data, suggesting the results, while consistent, should be interpreted with an understanding of the underlying experimental variability.
Discussion
Chemotherapy remains a cornerstone in cancer treatment, but it faces significant challenges like limited tumor specificity and multidrug resistance (MDR). Utilizing nanotechnology for targeted drug delivery presents a novel strategy to address these issues. Niosome nanoparticles are a promising drug delivery platform because they can serve as drug reservoirs and regulate drug release by altering their composition [33]. Numerous studies have explored different niosome formulations containing chemotherapeutic agents [34-35]. For instance, Rajput et al. [34] developed a pH-sensitive multilamellar gold niosome that encapsulated both Akt-siRNA and thymoquinone. They tested its effectiveness on tamoxifen- resistant MCF7 breast cancer cells with high Akt expression. The results demonstrated that this formulation successfully knocked down Akt, thereby increasing the cells’ sensitivity to thymoquinone. In another study, N-lauryl glucosamine (NLG) was used as a targeting ligand for doxorubicin loaded niosomal nanoparticles. The size of particles and entrapment efficiency were calculated 150 nm and 90%, respectively [35]. The combination of curcumin with cisplatin and carboplatin has shown significant synergistic effects in treating various types of cancer. Several studies have demonstrated that this combination can enhance the efficacy of chemotherapy and reduce the side effects associated with these drugs. In non-small cell lung cancer (NSCLC), combining curcumin with carboplatin exhibited synergistic anti-cancer effects. This combination effectively inhibited tumor cell proliferation, invasion, and migration. Additionally, pro-apoptotic genes such as caspase-3 and caspase-9 were upregulated, while the anti-apoptotic gene Bcl-2 was downregulated, leading to increased cancer cell apoptosis [20]. In colorectal cancer, curcumin significantly increased the chemosensitivity of cancer cells to cisplatin and carboplatin. The combination resulted in enhanced cell death through mechanisms such as apoptosis and cell cycle arrest, and also reduced the expression of NF-κB, which is linked to drug resistance [36]. In hepatocellular carcinoma (liver cancer), curcumin combined with cisplatin showed synergistic anti-tumor activity. This was attributed to the suppression of NF-κB activity and changes in the expression of genes related to cell survival and apoptosis [37]. Recent studies indicate that combining curcumin with platinum-based drugs can significantly enhance the cytotoxic efficacy of these drugs against chemotherapy-resistant cancer cells. For instance, in one study, the use of polymeric micelles containing both curcumin and platinum drugs in drug-resistant cells markedly increased the synergistic effect between these agents, highlighting their potential to boost chemotherapy effectiveness [38]. Another study found that demethoxycurcumin, a derivative of curcumin, restored drug sensitivity in cisplatin-resistant lung cancer cells by downregulating resistance proteins such as ERCC1 and activating apoptotic pathways [39]. In research employing dual-responsive nanoparticles loaded with curcumin and platinum drugs, this combination not only improved anticancer efficacy but also reduced chemotherapy side effects, specifically in non-small cell lung cancer [40]. Further investigations demonstrated that sequential administration of curcumin and cisplatin in ovarian cancer cells increased the cytotoxic effects and helped to overcome drug resistance [41]. Overall, due to its capacity to modulate signaling pathways associated with drug resistance and enhance apoptosis in cancer cells, curcumin has been recognized as an effective chemosensitizer that can improve the impact of platinum drugs on resistant cells [42]. These findings suggest that curcumin, as an adjunct to chemotherapy, could play a crucial role in targeted cancer treatment by mitigating drug resistance. Overall, the combination of curcumin with cisplatin and carboplatin enhances chemotherapy efficacy by promoting cancer cell death and overcoming drug resistance, suggesting its potential as an effective adjunct therapy in cancer treatment. With the increasing population and the increasing complexity of diseases, including cancers, infections, and health problems, there is an urgent need for up-to-date technologies to help solve these issues. In addition to physical problems, mental health issues can also lead to disorders and complications that align with physical problems. Nevertheless, humans have always sought to treat various disorders and diseases [43-65]. Oral squamous cell carcinoma, a prevalent and aggressive form of cancer, poses a significant challenge due to its complex microenvironment, where epigenetic regulation plays a crucial role in tumor progression and resistance to treatment, necessitating a deeper understanding of its underlying mechanisms to develop effective therapeutic strategies [66]. The development of effective cancer treatments is hindered by the limited understanding of the complex interactions between tumor-derived exosomes, such as those enriched with miRNA-211a, and cancer cells, such as B16F10 cells, highlighting the need for innovative drug delivery strategies that can target and modulate these interactions to improve treatment outcomes [67]. The COVID-19 pandemic, a global health crisis that affected millions of lives worldwide, has been successfully contained and overcome through concerted international efforts, advancements in medical technology, and the resilience of humanity [68]. One of the front-line defenses against this challenge were the healthcare workers, particularly nurses, who bore the brunt of the stress and pressure, working tirelessly to care for the infected and save countless lives [69]. One of the up-to-date technologies like cochlear implants can significantly impact a person’s progress [70]. Technology has also played a crucial role in the diagnosis of MAC (Mycobacterium Avium Complex) and has made a significant contribution to its detection and management [71]. Artificial intelligence has taken major steps in the diagnosis, treatment, and care of the disease [72]. In this study, we investigated and compared the cytotoxic effects of cisplatin and carboplatin nanoliposomes, both combined with curcumin, on CAL 27 oral cancer cells. The results revealed important differences between the two formulations in terms of nanoparticle size, stability, encapsulation efficiency, drug release, and cytotoxicity. Cisplatin nanoliposomes had a smaller particle size (175 nm) and more uniform size distribution (PDI 0.33) compared to carboplatin nanoliposomes (187 nm, PDI 0.48), suggesting better formulation stability. Furthermore, cisplatin had a higher encapsulation efficiency (84.5%) than carboplatin (76.5%), indicating that a greater amount of cisplatin was successfully incorporated into the liposomal structure, potentially enhancing its therapeutic effect. When examining the drug release profile, both nanoliposomes demonstrated sustained release over 52 hours, with cisplatin nanoliposomes releasing approximately 22% of the cisplatin, compared to 28% for carboplatin. Under acidic conditions (pH 5.2), which mimic the tumor microenvironment, carboplatin exhibited a faster release rate (57%) than cisplatin (44%), which could make carboplatin more effective in acidic tumor tissues. In terms of cytotoxicity, both platinum drugs, when combined with curcumin, showed significant enhancement in killing CAL 27 cells. Cisplatin exhibited slightly greater cytotoxicity than carboplatin, especially at lower curcumin concentrations. The combination of 4 mM curcumin with either cisplatin or carboplatin produced synergistic effects, amplifying the cell death beyond what was observed with individual treatments. However, at higher curcumin concentrations (8 mM), an antagonistic effect was observed, reducing the overall cytotoxicity. This suggests that while curcumin enhances the therapeutic effect of platinum-based drugs at moderate doses, excessive amounts may interfere with their function, possibly due to competitive interactions within the cancer cells.
Acknowledgments
None.
Data Availability
The data used in this study are derived from previously
published articles and are therefore not applicable.
Approved by a Scientific Body
This manuscript is not part of any student thesis or study, and therefore, approval from a scientific body is not applicable.
Ethical Issues and Approval
This study did not involve any original data collection or human subjects, and therefore, ethical approval was not required.
Consent for Publication
The author has provided consent for publication.
Conflict of Interest
The author declares no potential conflicts of interest.
References
- The global incidence of lip, oral cavity, and pharyngeal cancers by subsite in 2012 Shield KD , Ferlay J, Jemal A, Sankaranarayanan R, Chaturvedi AK , Bray F, Soerjomataram I. CA: a cancer journal for clinicians.2017;67(1). CrossRef
- Global epidemiology of oral and oropharyngeal cancer Warnakulasuriya S. Oral Oncology.2009;45(4-5). CrossRef
- Prognostic Role of Circulating Tumor Cells during Induction Chemotherapy Followed by Curative Surgery Combined with Postoperative Radiotherapy in Patients with Locally Advanced Oral and Oropharyngeal Squamous Cell Cancer Inhestern J, Oertel K, Stemmann V, Schmalenberg H, Dietz A, Rotter N, Veit J, et al . PloS One.2015;10(7). CrossRef
- Preoperative simultaneous cisplatin- or carboplatin-based chemotherapy and radiotherapy for squamous cell carcinoma of the oral cavity Kirita T., Ohgi K., Tsuyuki M., Kamikaido N., Yamamoto K., Sugimura M.. Journal of Surgical Oncology.1996;63(4). CrossRef
- The side effects of platinum-based chemotherapy drugs: a review for chemists Oun R, Moussa YE , Wheate NJ . Dalton Transactions (Cambridge, England: 2003).2018;47(19). CrossRef
- Carboplatin versus cisplatin Lippens R. J.. Pediatric Hematology and Oncology.1990;7(2). CrossRef
- Cisplatin in cancer therapy: molecular mechanisms of action Dasari S, Tchounwou PB . European Journal of Pharmacology.2014;740. CrossRef
- Developments in liposomal drug delivery systems Maurer N., Fenske D. B., Cullis P. R.. Expert Opinion on Biological Therapy.2001;1(6). CrossRef
- The suitability of liposomes for the delivery of hydrophobic drugs - A case study with curcumin Kolter M, Wittmann M, Köll-Weber M, Süss R. European Journal of Pharmaceutics and Biopharmaceutics: Official Journal of Arbeitsgemeinschaft Fur Pharmazeutische Verfahrenstechnik e.V.2019;140. CrossRef
- Employment of enhanced permeability and retention effect (EPR): Nanoparticle-based precision tools for targeting of therapeutic and diagnostic agent in cancer Kalyane D, Raval N, Maheshwari R, Tambe V, Kalia K, Tekade RK . Materials Science & Engineering. C, Materials for Biological Applications.2019;98. CrossRef
- Liposomal doxorubicin as targeted delivery platform: Current trends in surface functionalization Makwana V, Karanjia J, Haselhorst T, Anoopkumar-Dukie S, Rudrawar S. International Journal of Pharmaceutics.2021;593. CrossRef
- Cisplatin and platinum drugs at the molecular level. (Review) Boulikas T, Vougiouka M. Oncology Reports.2003;10(6).
- Experimental and clinical reports on anti-inflammatory, antioxidant, and immunomodulatory effects of Curcuma longa and curcumin, an updated and comprehensive review Memarzia A, Khazdair MR , Behrouz S, Gholamnezhad Z, Jafarnezhad M, Saadat S, Boskabady MH . BioFactors (Oxford, England).2021;47(3). CrossRef
- Curcumin inhibits proliferation, invasion, angiogenesis and metastasis of different cancers through interaction with multiple cell signaling proteins Kunnumakkara AB , Anand P, Aggarwal BB . Cancer Letters.2008;269(2). CrossRef
- Curcumin enhances the effect of chemotherapy against colorectal cancer cells by inhibition of NF-κB and Src protein kinase signaling pathways Shakibaei M, Mobasheri A, Lueders C, Busch F, Shayan P, Goel A. PloS One.2013;8(2). CrossRef
- Akt is upstream and MAPKs are downstream of NF-κB in paclitaxel-induced survival signaling events, which are down-regulated by curcumin contributing to their synergism Bava SV , Sreekanth CN , Thulasidasan AKT , Anto NP , Cheriyan VT , Puliyappadamba VT , et al . The International Journal of Biochemistry & Cell Biology.2011;43(3). CrossRef
- Bioavailability of curcumin: problems and promises Anand P, Kunnumakkara AB , Newman RA , Aggarwal BB . Molecular Pharmaceutics.2007;4(6). CrossRef
- Robust Microfluidic Technology and New Lipid Composition for Fabrication of Curcumin-Loaded Liposomes: Effect on the Anticancer Activity and Safety of Cisplatin Hamano N, Böttger R, Lee SE , Yang Y, Kulkarni JA , Ip S, Cullis PR , Li SD . Molecular Pharmaceutics.2019;16(9). CrossRef
- Curcumin enhances the effect of cisplatin in suppression of head and neck squamous cell carcinoma via inhibition of IKKβ protein of the NFκB pathway Duarte VM , Han E, Veena MS , Salvado A, Suh JD , Liang L, Faull KF , Srivatsan ES , Wang M . Molecular Cancer Therapeutics.2010;9(10). CrossRef
- Curcumin sensitizes human lung cancer cells to apoptosis and metastasis synergistically combined with carboplatin Kang JH , Kang HS , Kim IK , Lee HY , HaJH , Yeo CD , Kang HH , Moon HS , Lee SH . Experimental Biology and Medicine (Maywood, N.J.).2015;240(11). CrossRef
- Synergistic Effects of New Curcumin Analog (PAC) and Cisplatin on Oral Cancer Therapy Semlali A, Beji S, Ajala I, Al-Zharani M, Rouabhia M. Current Issues in Molecular Biology.2023;45(6). CrossRef
- Curcumin-loaded nanoparticles induce apoptotic cell death through regulation of the function of MDR1 and reactive oxygen species in cisplatin-resistant CAR human oral cancer cells Chang P, Peng S, Lee C, Lu C, Tsai S, Shieh T, Wu T, et al . International Journal of Oncology.2013;43(4). CrossRef
- Curcumin analog, GO-Y078, induces HO-1 transactivation-mediated apoptotic cell death of oral cancer cells by triggering MAPK pathways and AP-1 DNA-binding activity Chien M, Shih P, Ding Y, Chen L, Hsieh F, Tsai M, Li P, Lin C, Yang S. Expert Opinion on Therapeutic Targets.2022;26(4). CrossRef
- Enhancing Cisplatin Delivery via Liposomal Nanoparticles for Oral Cancer Treatment Ghanbarikondori P, Bagheri R, Saberian E, et al . Indian J Clin Biochem.2024. CrossRef
- Preparation of 6-Mercaptopurine Loaded Liposomal Formulation for Enhanced Cytotoxic Response in Cancer Cells Jamal A, Asseri AH , Ali EMM , El-Gowily AH , Khan MI , Hosawi S, Alsolami R, Ahmed TA . Nanomaterials (Basel, Switzerland).2022;12(22). CrossRef
- Cisplatin and curcumin co-loaded nano-liposomes for the treatment of hepatocellular carcinoma Cheng Y, Zhao P, Wu S, Yang T, Chen Y, Zhang X, He C, et al . International Journal of Pharmaceutics.2018;545(1-2). CrossRef
- Curcumin activates DNA repair pathway in bone marrow to improve carboplatin-induced myelosuppression Chen X, Wang J, Fu Z, Zhu B, Wang J, Guan S, Hua Z. Scientific Reports.2017;7(1). CrossRef
- Curcumin-cisplatin chemotherapy: A novel strategy in promoting chemotherapy efficacy and reducing side effects Hussain Y, Islam L, Khan H, Filosa R, Aschner M, Javed S. Phytotherapy research: PTR.2021;35(12). CrossRef
- Combining curcumin (C3-complex, Sabinsa) with standard care FOLFOX chemotherapy in patients with inoperable colorectal cancer (CUFOX): study protocol for a randomised control trial Irving GR , Iwuji CO , Morgan B, Berry DP , Steward WP , Thomas A, Brown K, Howells LM . Trials.2015;16. CrossRef
- Liposomal co-delivery of curcumin and albumin/paclitaxel nanoparticle for enhanced synergistic antitumor efficacy Ruttala HB , Ko YT . Colloids and Surfaces. B, Biointerfaces.2015;128. CrossRef
- In vitro anti-cancer activity and pharmacokinetic evaluation of curcumin-loaded lipid nanocapsules Lollo G, Ullio-Gamboa G, Fuentes E, Matha K, Lautram N, Benoit J. Materials Science & Engineering. C, Materials for Biological Applications.2018;91. CrossRef
- Intercalation of curcumin into liposomal chemotherapeutic agent augments apoptosis in breast cancer cells Mahmoudi R, Hassandokht F, Ardakani MT , Karimi B, Roustazadeh A, Tarvirdipour S, Barmak MJ , Nikseresht M, et al . Journal of Biomaterials Applications.2021;35(8). CrossRef
- Effects of Cisplatin-Loaded Niosomal Nanoparticleson BT-20 Human Breast Carcinoma Cells Kanaani L, Javadi I, Ebrahimifar M, Ebrahimi Shahmabadi H, Akbarzadeh Khiyav A, Mehrdiba T. Asian Pacific journal of cancer prevention: APJCP.2017;18(2). CrossRef
- Overcoming Akt Induced Therapeutic Resistance in Breast Cancer through siRNA and Thymoquinone Encapsulated Multilamellar Gold Niosomes Rajput S, Puvvada N, Kumar BNP , Sarkar S, Konar S, Bharti R, et al . Molecular Pharmaceutics.2015;12(12). CrossRef
- Glucosamine-anchored doxorubicin-loaded targeted nano-niosomes: pharmacokinetic, toxicity and pharmacodynamic evaluation Pawar S, Shevalkar G, Vavia P. Journal of Drug Targeting.2016;24(8). CrossRef
- Curcumin-enhanced chemosensitivity of FDA-approved platinum (II)-based anti-cancer drugs involves downregulation of nuclear endonuclease G and NF-κB as well as induction of apoptosis and G2/M arrest Wang Y, Liu H, Su C. International Journal of Food Sciences and Nutrition.2014;65(3). CrossRef
- Antitumor effects of curcumin, alone or in combination with cisplatin or doxorubicin, on human hepatic cancer cells. Analysis of their possible relationship to changes in NF-kB activation levels and in IAP gene expression Notarbartolo M, Poma P, Perri D, Dusonchet L, Cervello M, D'Alessandro N. Cancer Letters.2005;224(1). CrossRef
- Dual-drug delivery of curcumin and platinum drugs in polymeric micelles enhances the synergistic effects: a double act for the treatment of multidrug-resistant cancer Scarano W, Souza P, Stenzel MH . Biomaterials Science.2015;3(1). CrossRef
- Demethoxycurcumin increases the sensitivity of cisplatin-resistant non-small lung cancer cells to cisplatin and induces apoptosis by activating the caspase signaling pathway Chen Y, Hong C, Chen X, Qin Z. Oncology Letters.2020;20(5). CrossRef
- Platinum complexes of curcumin delivered by dual-responsive polymeric nanoparticles improve chemotherapeutic efficacy based on the enhanced anti-metastasis activity and reduce side effects Chen Y, Chen C, Zhang X, He C, Zhao P, Li M, Fan T, Yan R, et al . Acta Pharmaceutica Sinica. B.2020;10(6). CrossRef
- Synergism from sequenced combinations of curcumin and epigallocatechin-3-gallate with cisplatin in the killing of human ovarian cancer cells Yunos NM , Beale P, Yu JQ , Huq F. Anticancer Research.2011;31(4).
- Curcumin effect on cancer cells' multidrug resistance: An update Keyvani-Ghamsari S, Khorsandi K, Gul A. Phytotherapy research: PTR.2020;34(10). CrossRef
- Prediction of Breast Cancer Recurrence With Machine Learning. In M. Khosrow-Pour, D.B.A. (Ed.), Encyclopedia of Information Science and Technology Owrang OMM , Schwarz G, Horestani FJ . Sixth Edition. Advance online publication.2025. CrossRef
- Ultrasound Waves Effect on the Proliferation of Fibroblast Cells: Collagen Type I Expression Hormozi Moghaddam Z, Mokhtari-Dizaji M, Nilforoshzadeh MA , Bakhshandeh M. Journal of Biomedical Physics and Engineering.2023. CrossRef
- Synergistic anticancer effects of doxorubicin and metformin combination therapy: A systematic review Jalali F, Fakhari F, Sepehr A, Zafari J, Sarajar BO , Sarihi P, Jafarzadeh E. Translational Oncology.2024;45. CrossRef
- Enhancing breast cancer diagnosis accuracy through genetic algorithm-optimized multilayer perceptron. Multiscale and Multidisciplinary Modeling Talebzadeh H, Talebzadeh M, Satarpour M, Jalali F, Farhadi B, Vahdatpour MS . Experiments and Design, 1-17.2024;:1-17.
- The AI Diagnostician: Improving Medical Diagnosis with Artificial Intelligence Farrokhi M, Taheri F, Adibnia E, Mehrtabar S, Rassaf Z, Tooyserkani SH , Rajabloo Y, et al . Kindle.2024;4(1):1-219.
- Investigating the mortality trend of gastrointestinal cancers in Babol, North Iran (2013-2021) Ebrahimi P, Karami M, Delavari S, Shojaie L, Hosseini-Berneti SH , Bayani F, Moghaddasi M, Babazade O, Nikbakht H. BMC gastroenterology.2024;24(1). CrossRef
- Batch adsorption of methyl tert-butyl ether (MTBE) from aqueous solution by combined CNT and zeolite Behzadnezhad A, Ebadi T, Taheri S, Kowsari E. ResearchGate.2024. CrossRef
- Beta lactam allergy and risk of surgical site infection: A systematic review and meta-analysis Salehi SA , Hajishah H, Amini MJ , Movahed F, Ebrahimi A, Rihani FSS , Dehbozorgi M, et al . Current Problems in Surgery.2024;61(11). CrossRef
- Ethical Considerations and Equipoise in Cancer Surgery Vakili-Ojarood M, Naseri A, Shirinzadeh-Dastgiri A, Saberi A, HaghighiKian SM , Rahmani A, Farnoush N, et al . Indian Journal of Surgical Oncology.2024;15(Suppl 3). CrossRef
- A comprehensive review of etiology, pathophysiology, epidemiology, and management of hair loss and its correlation with COVID-19 Faraz Changizi , Maryam Abdolmaleki , Mina Farjam , Laya Ohadi . International Journal of Medicine.2024;12(2):41-47. CrossRef
- The Effect of Pharmacist Interventions on the Antimicrobial Prevention Pattern in Vascular and Gastrointestinal Surgeries: A Prospective Study Yazd H, Ohadi L, Abdolmaleki M, Farsi Y, Pishgahi M. J Case Rep Med Hist.2024;4(4). CrossRef
- Serum level measurement of progranulin in relapsing-remitting multiple sclerosis and neuromyelitis optica patients Azadani NN , Norouzi F, Hajizadeh M, Parsa S, Khalighinejad F. American Journal of Clinical and Experimental Immunology.2019;8(3).
- Comparing the effect of sedation with dexmedetomidine and propofol on sleep quality of patients after cardiac surgery: A randomized clinical trial Azarfarin R, Ziaei Fard M, Ghadimi M, Chaibakhsh Y, Yousefi M. Journal of Cardiovascular and Thoracic Research.2024;16(3). CrossRef
- A Comprehensive Review of Electromyography in Rehabilitation: Detecting Interrupted Wrist and Hand Movements with a Robotic Arm Approach Savoji K, Soleimani M, Moshayedi AJ . EAI Endorsed Transactions on AI and Robotics.2024;3. CrossRef
- Pseudoaneurysm formation after placement of a FRED flow diverter stent in a patient with iatrogenic ICA injury during transsphenoidal surgery: A case report Hasanpour M, Golchin N, Mirsardoo H, Alagha A, Elyassirad D, Keykhosravi E. Neuro-Chirurgie.2024;70(1). CrossRef
- Flow diverting stent monotherapy as the best choice in the treatment of intracranial blood blister-like aneurysms: a systematic review Ghorbani M, Keykhosravi E, Vatanparast M, Elyassirad D, Kakhki FT , Gheiji B, Golchin N, et al . Neurosurgical Review.2024;47(1). CrossRef
- Advances in Technical Assessment of Spiral Inertial Microfluidic Devices Toward Bioparticle Separation and Profiling: A Critical Review Bagi M, Amjad F, Ghoreishian SM , Sohrabi Shahsavari S, Huh YS , Moraveji MK , Shimpalee S. ResearchGate.2024. CrossRef
- Scaffold Application for Bone Regeneration with Stem Cells in Dentistry: Literature Review Saberian E, Jenča A, Zafari Y, Jenča A, Petrášová A, Zare-Zardini H, Jenčová J. Cells.2024;13(12). CrossRef
- Comparative Analysis of Osteoblastic Responses to Titanium and Alumina-Toughened Zirconia Implants: An In Vitro Study Saberian E, Jenča A, Seyfaddini R, Jenča A, Zare-Zardini H, Petrášová A, Jenčová J. Biomolecules.2024;14(6). CrossRef
- Enhancing Cisplatin Delivery via Liposomal Nanoparticles for Oral Cancer Treatment | Request PDF Ghanbarikondori P, Aliakbari RBS , Saberian E, Jenča A, Petrášová A, Jenčová J, et al . Indian Journal of Clinical Biochemistry.2024. CrossRef
- Examine the impact of mental planning on building mental health and cognitive beliefs of bank employees Ghahjavarestani AM . ResearchGate.2024. CrossRef
- Redictive Role of Personality Dimensions on Quality of Life and Satisfaction in Patients with Gender Identity Disorder after Gender Reassignment Surgery Ghahjavarestani AHM . The scientific heritage .2024. https://orcid. org/0000-0002-0440-0509;135.
- Plasma NT1 tau is associated with hypometabolism in Alzheimer’s disease continuum. Neurology Letters, 3(Special Issue (Diagnostic and Therapeutic advances in Neurodegenerative diseases)) Ghahri Lalaklou Z, Montazeri Ghahjavarestani A, Pishkari Y, Emami D. 2024;:8-13. CrossRef
- Epigenetic Regulation in Oral Squamous Cell Carcinoma Microenvironment: A Comprehensive Review Mesgari H, Esmaelian S, Nasiri K, Ghasemzadeh S, Doroudgar P, Payandeh Z. Cancers.2023;15(23). CrossRef
- The effects of tumor-derived exosomes enriched with miRNA-211a on B16F10 cells Atashzar M, Ataollahi M, Asad A, Doroudgar P, Amani D. Contemporary Oncology (Poznan, Poland).2024;28(2). CrossRef
- A Multistage Stochastic Optimization Model for Resilient Pharmaceutical Supply Chain in COVID-19 Pandemic Based on Patient Group Priority. 2024 Systems and Information Engineering Design Symposium (SIEDS) Mahdavimanshadi M, Anaraki MG , Mowlai M, Ahmadirad Z. IEEE. .2024;:382-387. CrossRef
- A qualitative approach to the ethical challenges of Iranian nurses during the COVID-19 pandemic Rajabipoor Meybodi A, Mohammadi M, Arjmandi H. Journal of Preventive and Complementary Medicine.2022;1(3):156-162. CrossRef
- Evaluating the effect of cochlear implantation age on pragmatic abilities before and after age of 3 Nikrah P, Ghareh Chahie R, Ghazvini A, Hajizadeh A. Applied Neuropsychology. Child.2024. CrossRef
- Caseous calcification of the mitral annulus presenting with symptomatic complete heart block Nikoo M, Danesh S, Abtahi F. Journal of Clinical Images and Medical Case Reports.2022;3. CrossRef
- Examination of AI’s role in Diagnosis, Treatment, and Patient care. In Gupta, M., Kumar, R., & Lu, Z. (Eds.), Transforming Gender-Based Healthcare with AI and Machine Learning Sajjadi Mohammadabadi SM , Seyedkhamoushi F, Mostafavi M, Borhani Peikani M. CRC Press.2024;:(1st ed., pp. 221- 238). CrossRef
License

This work is licensed under a Creative Commons Attribution-NonCommercial 4.0 International License.
Copyright
© Asian Pacific Journal of Cancer Biology , 2024
Author Details