Minimizing the Risk of Sample Mix-ups in the Molecular Pathology Section in Oncology Center Using Risk Assessment Matrix (RAM)
Download
Abstract
Background: Sample mix-ups in molecular pathology can result in diagnostic errors, inappropriate treatment, and compromised patient safety. These risks are exacerbated in oncology settings, where accurate diagnoses directly impact patient outcomes.
Purpose: This study aims to evaluate the effectiveness of the Risk Assessment Matrix (RAM) in minimizing the risk of sample mix-ups in the molecular pathology section of an oncology center.
Methods: A prospective quality improvement design was adopted, comparing pre- and post-intervention data to assess the impact of RAM. Risks were identified through quality rounds and categorized using the RAM, where Likelihood (L) and Severity (S) scores were assigned to each risk (L × S = Risk Score). Interventions included automation, barcode labeling, revised workflows, and staff training. The effectiveness of interventions was measured through re-evaluation of risk scores and percentage risk reduction.
Results: The interventions resulted in significant improvements across multiple areas. The risk score for Excel-based registration dropped from 16 to 2, representing an 88% reduction. Handwritten labeling errors decreased by 83%, and inaccurate documentation in LIS/HIS systems was reduced by 83%. Additionally, the risk associated with unattended PTS sample transport was lowered by 63%, and eliminating manual entry processes reduced errors by 67%. Most risks showed reductions above 60%, demonstrating the effectiveness of RAM in improving sample management and patient safety.
Conclusion: The application of RAM in the molecular pathology section of an oncology center significantly reduced the likelihood of sample handling errors, enhancing both diagnostic accuracy and patient safety. The study highlights the importance of automation, real-time monitoring, and multidisciplinary collaboration in sustaining these improvements. RAM provides a structured framework for prioritizing and mitigating risks in complex healthcare workflows.
Introduction
Sample mix-ups are a significant concern in molecular pathology and oncology, leading to diagnostic errors, inappropriate treatment decisions, and compromised patient safety [1]. The risk of such mix-ups is heightened by the complexity of molecular diagnostics, which often requires intricate workflows, including manual handling, multiple processing steps, and reliance on accurate data entry [2]. In oncology settings, where precise diagnosis directly influences patient care plans, any misidentification of samples can have severe clinical and financial implications [3].
Sample mix-ups can have devastating consequences for patients, particularly in oncology. Diagnostic errors stemming from misidentified samples may lead to incorrect or delayed treatment, such as administering therapies that are either ineffective or harmful [2, 5]. For instance, a patient with an undiagnosed aggressive cancer subtype could receive a less intensive treatment, allowing the disease to progress unchecked. Conversely, a patient misdiagnosed with cancer may endure unnecessary treatments with severe side effects [2, 3, 4].
Beyond the immediate clinical ramifications, sample mix-ups can undermine patient trust in the healthcare system and result in long-term psychological distress [1, 3]. Misdiagnoses can also have a ripple effect on clinical trials and research, as inaccurate data compromises study integrity. Addressing these risks through robust sample handling protocols is essential to maintaining high standards of patient care and advancing oncological research [3, 5].
Sample mix-ups carry significant legal and regulatory consequences, as they directly impact patient safety and the quality of care [3, 5]. Healthcare institutions may face malpractice lawsuits, financial penalties, or loss of accreditation if such errors result in harm to patients [5]. Regulatory bodies, such as JCI or national healthcare authorities, mandate stringent sample handling protocols, and any deviations may trigger compliance audits or sanctions [2, 5, 6].
Effective risk management practices, such as implementing a Risk Assessment Matrix (RAM), are essential to reduce the likelihood of sample handling errors. RAM is a systematic tool designed to identify, assess, and prioritize risks based on their potential impact and likelihood, enabling targeted mitigation strategies [6-8].
Incorporating RAM in molecular pathology laboratories facilitates early identification of potential errors, ensuring proactive measures to prevent sample mix-ups [8]. This process involves a collaborative approach among pathologists, laboratory staff, and quality teams, leading to robust controls, such as automation and standardized protocols [9]. Regular quality audits are essential to monitor these practices and ensure that risk management strategies remain effective and aligned with technological advancements [10, 11].
This study investigates the effectiveness of using a Risk Assessment Matrix (RAM) to minimize the risk of sample mix-ups in the molecular pathology section of an oncology center. By systematically identifying high-risk practices and implementing targeted interventions, RAM aims to enhance diagnostic accuracy, improve patient outcomes, and uphold patient safety standards [6, 10, 11].
Methods
Study Design
This study employed a prospective quality improvement design focused on implementing the Risk Assessment Matrix (RAM) to minimize sample mix-ups in the molecular pathology section of an oncology center. The design emphasized identifying high-risk practices, prioritizing risks, and applying targeted interventions [6, 10, 11]. The study followed a pre-and post-intervention approach, comparing data from before and after RAM implementation to measure its effectiveness.
The effectiveness of mitigation strategies is measured through pre- and post-intervention analyses using tools like RAM. Key performance indicators (KPIs), such as error rates, turnaround times, and sample rejections, provide quantifiable metrics to evaluate improvements. Regular audits, random sample checks, and process observations are also conducted to monitor adherence to revised protocols and identify areas requiring further refinement [6].
Quality improvement teams analyze the data collected during monitoring to ensure sustained effectiveness. Staff feedback is another critical component, offering insights into the practicality and challenges of implemented strategies. These measures collectively ensure that risk mitigation efforts remain effective and responsive to changing conditions [12-15].
Setting
The study was conducted in the molecular pathology section of a specialized oncology center in Oman. The facility provides diagnostic and molecular testing services for cancer diagnosis and treatment planning. The setting involved collaboration across departments, including molecular diagnostics, pathology, and quality assurance, ensuring the comprehensive risk management framework covering the entire sample handling process. The center typically establishes clear reporting channels and protocols for sample mix-ups. Incident reporting systems enable staff to document errors promptly, and root cause analyses (RCAs) are conducted to identify underlying issues. These investigations involve multidisciplinary teams to ensure a comprehensive understanding of the incident and develop targeted corrective actions. Findings from RCAs are often shared during team meetings or training sessions to reinforce learning and prevent recurrence. Institutions may also implement real-time monitoring systems, such as automated alerts, to detect and address errors as they occur.
RAM Methods
Team formulation
A multidisciplinary team consisting of experts from quality management, laboratory quality control, and nursing was formed to carry out this task. This diverse team ensured that risks were identified from multiple perspectives, capturing insights across clinical, operational, and quality dimensions.
Process Identification
The Risk Assessment Matrix (RAM) process began by identifying processes for assessment within the molecular pathology section, focusing on workflows where sample handling errors were likely to occur. Accordingly flow chart was developed.
The workflow for handling and processing samples in the molecular pathology section involves multiple stages, beginning with nursing staff and moving through lab orderly staff and molecular staff, each playing a critical role. The process starts with nurses checking patient orders and printing labels one by one for the tests. For tests that use the same tube, free labels are printed to avoid duplication, and extra labels are prepared and logged in a book to track the samples being sent to the lab. Once labeled, the samples are sent through nursing channels to the lab for further processing.
Upon arrival at the lab, a medical orderly receives the samples, consolidating them from different sources, even if they contain free labels. These samples are then registered in Excel, grouped according to their section, and prepared for delivery to the molecular section. The samples are often unattended on trolleys and sent to the molecular lab for further registration and testing.
Once in the molecular lab, the molecular staff handles the registration and labeling process. They copy and paste sample information into labeling sheets and register the samples in the Laboratory Information System (LIS) to log their arrival. The same staff manually numbers the tubes by hand, adding another labeling layer. Additionally, they complete sample registration by copying data into the hospital information system (HIS) using Excel sheets, ensuring that all records align across systems. Once registration is complete, the samples are stored in a refrigerator until they are ready for testing.
During the testing phase, molecular staff organize samples in the refrigerator by placing each patient’s samples in one row, according to the list. Witness verification is required for specific tests while loading the samples into plates. The plates are loaded into the machine for testing, following the correct order. In some cases, tubes and plates are prepared using LIS labels to ensure traceability. Once the tests are complete, the plates are unloaded from the machine, again with witness verification for select tests, to ensure accountability.
Throughout the process, several potential risks emerge. These include using unattended trolleys, increasing the chance of misplacement, and manual handwriting on tubes, which can lead to labeling errors. The reliance on copy-pasting between multiple systems (Excel, LIS, HIS) adds further risk for transcription mistakes. Witness verification is applied inconsistently, leaving gaps in oversight. Additionally, free labeling without standardization creates confusion, and the manual nature of many steps introduces further opportunities for human error.
Risk Identification
This step involved risk identification through multidisciplinary quality rounds. A team comprising pathologists, laboratory staff, and quality assurance personnel thoroughly evaluated the molecular pathology processes. During these rounds, the team identified vital points where errors were likely to occur, such as improper labeling of samples, contamination during handling, and manual data entry mistakes (2). The team reviewed historical incident reports to ensure a comprehensive risk profile and interviewed staff to gather additional insights on past errors and challenges (Table 1).
Risk identification | Evaluation | Intervention | RE-Evaluation | Differences in L*S | (%) | ||||
Main Risk | L | S | L*S | L | S | L*S | |||
Printing extra labels indicating sending samples | 3 | 2 | 6 | Discontinue the use of extra labels; replace them with electronic tracking systems. | 2 | 1 | 2 | 4 | 67 |
Samples sent through PTS unattended | 4 | 4 | 16 | Implement secure transport protocols with tamper-evident containers and monitoring systems. | 2 | 3 | 6 | 10 | 63 |
Combining samples from different sources with free labels | 4 | 4 | 16 | Ensure separate handling and labeling for samples from different sources; use an integrated LIS to eliminate free labels. | 2 | 3 | 6 | 10 | 63 |
Registration in Excel and handwriting section sample numbers | 4 | 4 | 16 | Transition to using LIS for all registration and labeling processes, eliminating Excel usage. | 1 | 2 | 2 | 14 | 88 |
Manual entry of sample details into the LIS system | 3 | 4 | 12 | Standardize electronic data entry procedures; implement double-checking and validation processes to minimize errors. | 2 | 2 | 4 | 8 | 67 |
Handwriting labels on all tubes | 4 | 3 | 12 | Eliminate handwritten labels by using automated label printers integrated with LIS. | 1 | 2 | 2 | 10 | 83 |
Nursing non-compliance with ID identification (two identifiers) | 4 | 4 | 16 | Implement strict adherence to ID policies with education, enforcement, and disciplinary actions; double-check the system for all sample handling. | 2 | 3 | 6 | 10 | 63 |
Loading/unloading samples without proper witness | 2 | 3 | 6 | Establish a formalized witness protocol for critical steps; implement electronic verification where possible. | 1 | 2 | 2 | 4 | 67 |
Incomplete patient and sample location data | 3 | 3 | 9 | Ensure the LIS system includes comprehensive patient and sample location information; require mandatory fields for critical data entries. | 1 | 2 | 2 | 7 | 78 |
Inaccurate documentation in HIS/LIS | 3 | 4 | 12 | Enforce accurate documentation protocols, including clear guidelines for data entry in HIS and LIS, and restrict unauthorized edits. | 1 | 2 | 2 | 10 | 83 |
Risk Assessment and Prioritization
After identifying potential risks, the study team developed a customized Risk Assessment Matrix (RAM) to evaluate and prioritize them systematically. Each risk was assessed using two key dimensions: Likelihood of Occurrence (L) and Impact on Patient Safety and Diagnostic Accuracy (S). Likelihood (L) was rated on a scale from 1 (Rare) to 5 (Almost Certain), reflecting the probability of the error occurring based on historical data and expert judgment. Similarly, Severity (S) was rated from 1 (Insignificant) to 5 (Catastrophic), based on the potential consequences the error could have on patient safety and diagnostic outcomes.
To prioritize risks, the RAM calculated a risk score by multiplying these two values (L × S = Risk Score), with the product ranging from 1 to 25. Risks were categorized into low (1–6), medium (7–14), or high priority (15–25). This matrix visually represented all identified risks, helping the team focus their efforts on those with the highest scores. High-priority risks required immediate interventions, while medium and low-priority risks were managed with standard precautions and periodic monitoring. This prioritization ensured that resources were directed toward mitigating the most critical risks first, enhancing patient safety and diagnostic reliability (Table 1).
Control Measures and Mitigation Strategies
For high-priority risks, the team developed and implemented targeted mitigation strategies based on previous analysis and extensive literature (10, 13-21), including:
• Standardizing labeling protocols: Barcode and advance electronic systems were introduced to reduce human errors associated with manual labeling.
• Staff training and education: Laboratory personnel received ongoing training sessions emphasizing correct sample handling and data entry practices. Staff training programs focused on best practices in sample handling, including proper labeling, accurate data entry, and strict compliance with identification protocols. Training sessions incorporated hands-on demonstrations, role-playing scenarios, and updates on new technologies and procedures. Regular competency assessments ensured that staff maintained proficiency in these critical areas. The training also emphasized interdisciplinary collaboration, fostering open communication among nurses, lab personnel, and quality teams.
• Automation: Automated systems were incorporated to reduce reliance on manual data entry, thereby minimizing the risk of transcription errors
• Revised protocols: New workflows were developed for sample receipt, processing, and storage, ensuring greater consistency and compliance with quality standards.
• Improving Awareness of Sample Mix-Up Risks: Efforts to raise awareness about sample mix-up risks included education campaigns, workshops, and visual aids such as posters and flowcharts displayed prominently in laboratories. Data on error rates and real-life case studies were shared during staff meetings, highlighting the importance of following protocols. Incorporating sample mix-up scenarios into training programs further prepared staff to recognize and mitigate potential risks. Leadership actively championed these awareness initiatives by promoting safety campaigns and recognizing staff for adherence to safety protocols.
• Past incidents of sample mix-ups highlighted the critical role of automation, standardization, and communication in reducing errors. These events revealed gaps in workflows, such as reliance on manual processes and the absence of witness verification. Lessons learned underscored the importance of continuous monitoring, regular updates to protocols, and proactive staff training to address these vulnerabilities. Corrective actions based on past incidents, such as adopting barcode systems and revising workflows, were implemented to prevent similar errors from recurring. Sharing these lessons across departments fostered a unified approach to risk management, ensuring consistent adherence to improved practices.
These interventions were designed to address the identified risks directly and improve the reliability of molecular pathology processes (Table 1).
Risk Re-Evaluation After Intervention
Following implementing the mitigation strategies, the team conducted a risk re-evaluation. The risks were reassessed using the same RAM framework to determine whether the likelihood and impact had decreased. This step ensured that the interventions effectively reduced risks to acceptable levels. If any risks remained high, additional adjustments to the interventions were considered (Table 1).
Monitoring and Quality Audits
To maintain the effectiveness of the risk management process, monitoring and quality audits were conducted at regular intervals, ensuring that interventions were consistently applied and practical. These audits included random sample checks to detect ongoing or new errors, process observations to verify staff compliance with revised protocols, and staff feedback to uncover practical challenges and refine workflows as needed. Key performance metrics, such as error rates and the frequency of sample handling incidents, were tracked to assess progress. Data from these audits provided valuable insights into the impact of the interventions, guiding continuous improvements and ensuring the sustainability of risk management efforts.
The Risk Assessment Matrix (RAM) was updated through regular reviews that incorporated findings from audits, staff feedback, and lessons learned from past incidents. Technological advancements, such as improved barcode systems or new laboratory equipment, were reflected in the matrix by adjusting risk likelihood and severity scores to align with updated workflows. Scoring criteria were revised to match current standards, and changes were integrated into staff training to ensure consistency and understanding across teams. Cross-departmental collaboration captured the full impact of new processes, ensuring the RAM remained an effective tool for prioritizing and mitigating risks in an evolving healthcare environment.
Ethical Considerations
The study adhered to ethical guidelines for quality improvement projects. All data collected, including incident reports and staff interviews, were anonymized to protect confidentiality. Ethical approval was obtained from the hospital’s research ethics committee, ensuring the interventions aligned with institutional policies and did not compromise patient care (CCCRC-102-2024).. Staff participation was voluntary, and feedback was collected anonymously to ensure honest reporting without fear of reprisal.
Results
Several critical risks were identified with varying likelihood (L) and severity (S). For example, printing extra labels for tracking samples had a moderate risk score of 6 (L=3, S=2), while unattended sample transport through PTS (Pneumatic Tube System) and mixing samples with free labels were both rated at 16 (L=4, S=4) due to their significant impact on patient safety. Risks related to manual registration and handwritten labeling were also highly ranked, scoring 12-16, reflecting the vulnerabilities of these manual processes (Table 1).
After targeted interventions were implemented, the re-evaluation of risks showed significant improvements. For instance, discontinuing extra labels and adopting electronic tracking systems reduced the risk score from 6 to 2, achieving a 67% reduction. Similarly, implementing secure transport protocols for PTS reduced its score from 16 to 6 (a 63% reduction), and ensuring separate handling for samples with integrated LIS tracking reduced the combined sample risk from 16 to 6 (also a 63% reduction) (Figure 1).
Figure 1. Flow Chart.
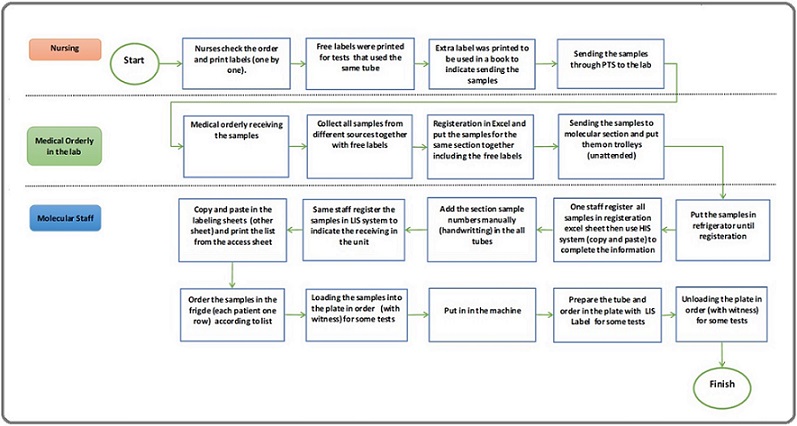
Eliminating manual processes moving from Excel- based registration to a fully integrated LIS and replacing handwritten labels with automated printers resulted in some of the highest reductions. For example, transitioning from Excel registration reduced the score from 16 to 2, achieving an 88% reduction, while replacing handwritten labels with automated printing cut the risk from 12 to 2, representing an 83% reduction.
Risks related to nursing non-compliance with patient ID verification and loading/unloading samples without a witness were also mitigated. After implementing strict ID policies and formal witness protocols, these risks were reduced by 63% and 67%, respectively. Similarly, ensuring complete patient/sample location data reduced the risk from 9 to 2 (a 78% reduction), and reinforcing documentation practices in HIS/LIS systems achieved an 83% reduction in documentation errors.
Figures 2 and 3 illustrate the percentage reductions in risk scores achieved through targeted interventions across critical areas in the molecular pathology workflow.
Figure 2. Risk Reduction.
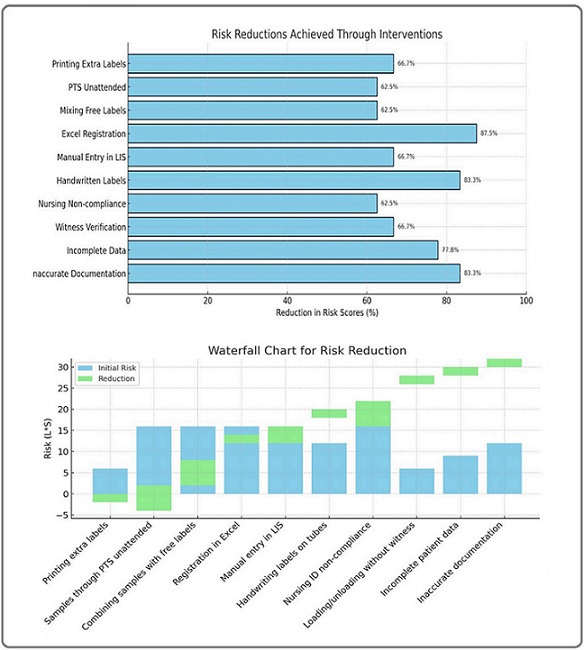
Figure 3. Intervention Impact on Risks.
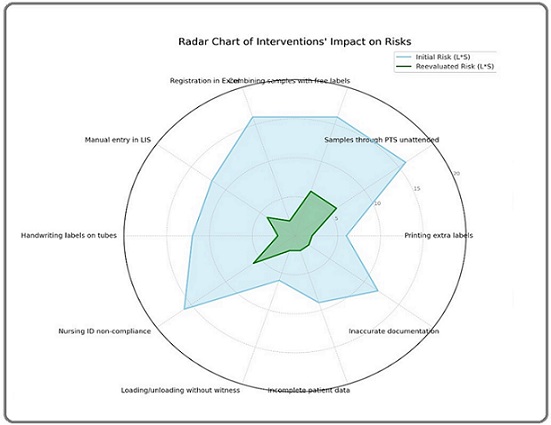
The most significant improvement was seen in Excel registration, with an 87.5% reduction, reflecting the positive impact of transitioning to an integrated LIS system. Handwritten labels and inaccurate documentation also showed substantial reductions of 83.3%, demonstrating the effectiveness of automating labeling processes and enforcing stricter documentation protocols. Addressing incomplete data achieved a 77.8% reduction by ensuring mandatory data fields in the LIS system. Risks associated with PTS transport and mixing free labels were reduced by 62.5% each by implementing secure handling protocols and integrated labeling systems. Similarly, interventions targeting nursing non-compliance and witness verification resulted in 63-67% reductions, reinforcing the importance of adherence to patient ID policies and proper sample handling procedures. Overall, the figure highlights the effectiveness of the interventions, with most risks reduced by more than 60%, significantly enhancing patient safety and process reliability.
The radar chart analysis of the implemented interventions demonstrated a significant reduction in risk levels across multiple categories (Figure 3). Each axis represented distinct risks, such as “Printing extra labels” and “Manual entry in LIS,” with the initial risk levels depicted by the blue-shaded area and reevaluated risks by the green-shaded area. The smaller size of the green area clearly indicated the effectiveness of interventions, such as transitioning to the Laboratory Information System (LIS) and using automated label printers, in reducing risks and improving sample management reliability. Overall, the chart effectively highlighted the success of these interventions, pinpointing where improvements were most impactful and identifying areas for further attention.
Discussion
This study explored the application of a Risk Assessment Matrix (RAM) to minimize sample mix-ups in the molecular pathology section of an oncology center. Sample mix-ups are a severe challenge in molecular diagnostics, where errors can result in inappropriate treatments, delayed diagnoses, and patient harm [1, 3]. Given the complexity of molecular workflows that rely on manual handling, multiple processing steps, and data entry, the risk of such errors is inherently high [2]. Addressing these risks through effective management strategies is essential to maintaining diagnostic accuracy and patient safety [1, 6].
The RAM framework was pivotal in identifying and prioritizing risks, ensuring resources were focused on the most critical vulnerabilities [16, 17]. This systematic approach was particularly relevant in a specialized oncology setting, where minor errors can significantly affect patient care [7]. The initial quality rounds and multidisciplinary team efforts provided a detailed overview of the main risks, such as improper labeling, manual data entry mistakes, and inconsistent handling protocols, highlighting the need for targeted interventions [7, 18].
A key strength of this study was forming a multidisciplinary team involving pathologists, laboratory quality control personnel, and nursing staff [9, 15]. This collaboration ensured a holistic evaluation of workflows and allowed multiple perspectives to inform the risk mitigation strategies [3]. As noted in prior research, collaboration between clinical and quality teams is critical to developing sustainable risk management practices [9, 13].
The implementation of RAM enabled a structured evaluation of each risk based on likelihood and severity (L × S), with risks categorized as low, medium, or high priority. This prioritization focused on critical risks such as unattended sample transport and manual labeling, which could significantly impact patient safety [2, 19]. For example, risks associated with manual Excel registration and free labeling scored high initially, underscoring the need for automation and electronic tracking interventions [2, 20].
Control measures were implemented for high-priority risks, with interventions such as barcode systems for labeling, automated data entry, and new workflows for sample processing. The study findings align with prior research, showing that automation and standardization effectively reduce manual errors and improve workflow efficiency [6, 9]. Furthermore, staff training and education were emphasized to ensure consistent adherence to updated protocols, reinforcing the importance of continuous education in maintaining quality [3, 7].
The impact of these interventions was significant, as demonstrated by the re-evaluation scores. For example, introducing electronic tracking systems reduced the risk score for labeling issues from 6 to 2, a 67% reduction. Similarly, implementing secure transport protocols for the Pneumatic Tube System (PTS) reduced the risk score from 16 to 6, reflecting a 63% reduction. These results validate the effectiveness of targeted interventions in mitigating high-risk practices [11].
For high-priority risks, targeted mitigation strategies were implemented to address critical vulnerabilities in the molecular pathology workflow. Standardizing labeling protocols by introducing barcode systems significantly reduced human errors associated with manual labeling. This automation enhanced the accuracy and traceability of sample identification [6, 14]. Staff training programs played a pivotal role in reinforcing best practices, incorporating hands-on demonstrations, role-playing, and updates on new technologies. By emphasizing proper labeling, accurate data entry, and interdisciplinary collaboration, these sessions ensured that personnel across departments worked cohesively to minimize risks. Regular competency assessments further sustained staff proficiency, fostering a culture of accountability and continuous improvement [19, 20].
Automation emerged as a cornerstone of error prevention, effectively minimizing transcription errors associated with manual data entry. Automated systems streamlined sample registration, labeling, and tracking, reducing the likelihood of mix-ups and enhancing overall workflow efficiency [4, 12, 19]. In addition to automation, revised protocols for sample receipt, processing, and storage were established to ensure consistency and compliance with quality standards [3, 6]. These protocols addressed previously identified gaps, such as the absence of witness verification and reliance on manual processes [19, 20]. The integration of structured workflows not only mitigated high-priority risks but also promoted adherence to established quality benchmarks [5, 17].
Raising awareness of sample mix-up risks was another critical component of the mitigation strategies. Education campaigns, workshops, and visual aids, such as posters and flowcharts, reinforced the importance of adhering to safety protocols [11-13]. Sharing error rates and real-life case studies during staff meetings helped contextualize the impact of errors and motivated adherence to best practices. Leadership support further strengthened these efforts, with safety campaigns and recognition programs fostering a proactive culture of risk management [4-6]. Lessons from past incidents of sample mix-ups informed these strategies, highlighting the importance of automation, standardization, and communication. Corrective actions, including workflow revisions and departmental collaboration, ensured that improvements were both sustained and integrated across the organization [1, 18].
Automation played a central role in many of the risk mitigation strategies. By eliminating handwritten labels and manual data entry, the study achieved some of the highest reductions in risk scores, with improvements of 83% for handwritten labels and 88% for Excel registration. This aligns with findings from other studies highlighting the importance of integrated systems like LIS in reducing transcription errors and ensuring data consistency [3, 21]. The study also highlighted the value of witness verification protocols for critical steps in sample handling. Establishing formal witness verification processes resulted in a 67% reduction in associated risks. Additionally, enforcing nursing compliance with patient identification policies reduced risks by 63%, reflecting the importance of strict policy adherence in minimizing identification errors [1, 5, 20].
Another area where significant improvements were observed was ensuring complete patient and sample location data. Integrating comprehensive data fields within the LIS system reduced the risk score for incomplete data from 9 to 2, a 78% reduction. The study’s emphasis on accurate documentation protocols further reduced the risk of errors in the HIS and LIS systems by 83% [11, 20]. These improvements demonstrate how systematic documentation practices contribute to enhanced process reliability [11, 15].
The monitoring and quality audits conducted throughout the study played a crucial role in sustaining the effectiveness of the interventions. Regular audits, including random sample checks, process observations, and staff feedback, ensured that risks were continuously monitored and managed [10, 13]. The use of key performance metrics, such as error rates and sample handling incidents, provided valuable insights into the impact of the interventions, allowing the team to make data-driven adjustments and ensure continuous improvement [5, 7].
The study also adhered to ethical standards by ensuring anonymity in data collection and obtaining approval from the hospital’s research ethics committee. The focus on staff participation and anonymous feedback facilitated open communication, enabling honest reporting of challenges and supporting process refinements [4, 6, 8].
The results of this study provide strong evidence that the RAM framework is an effective tool for minimizing sample mix-ups in molecular pathology. The observed reductions in risk scores, ranging from 63% to 88%, demonstrate the significant impact of targeted interventions on improving workflow efficiency and patient safety. For example, transitioning to barcode systems and automated printers was instrumental in reducing labeling errors, while secure transport protocols addressed risks associated with sample movement [22, 23]. These findings are consistent with prior research, reinforcing the importance of standardization, automation, and policy enforcement in healthcare settings [22, 23].
Limitation
One fundamental limitation of the study is the reliance on manual audits and staff feedback for monitoring. While these methods provided valuable insights, incorporating real-time electronic monitoring systems could enhance the sustainability of the risk management process. Additionally, the study focused primarily on internal workflows; external factors, such as third-party sample transportation risks, were not addressed, representing an area for future improvement.
Clinical Implications
The clinical implications of minimizing sample mix-ups in molecular pathology are profound, particularly in oncology, where precision is paramount. Accurate sample handling directly affects diagnostic accuracy, enabling timely and appropriate treatment planning for patients. By implementing RAM-based interventions, oncology centers can enhance diagnostic reliability, reducing the risk of inappropriate treatments, delayed interventions, and potential harm to patients. Improved sample handling also fosters trust between patients and healthcare providers, which is crucial in settings where outcomes are closely tied to accurate diagnoses.
Additionally, reducing sample mix-ups can lead to improved workflow efficiency and resource utilization. By automating processes and standardizing protocols, laboratories can minimize errors that require re-testing or additional investigations, reducing the financial burden on both the healthcare system and patients. These improvements also alleviate stress on laboratory staff, promoting a safer and more productive working environment that prioritizes patient care and safety.
In conclusion, this study demonstrates that implementing a Risk Assessment Matrix (RAM) in the molecular pathology section of an oncology center significantly reduces the risk of sample mix-ups, improving diagnostic accuracy and patient safety.
The findings reveal that targeted interventions such as automation, barcode systems, secure transport protocols, and revised workflows effectively address high-risk practices. The overall reduction in risk scores, ranging from 63% to 88%, reflects the success of a systematic approach to risk management. The multidisciplinary collaboration, continuous monitoring, and proactive protocol adjustments have contributed to maintaining high-quality standards in sample handling. These results reinforce the value of RAM as an essential tool for improving healthcare processes and minimizing the potential for errors in complex workflows.
Acknowledgments
Statement of Transparency and Principals:
• Study was approved by Research Ethic Committee of author affiliated Institute.
• Study’s data is available upon a reasonable request.
• All authors have contributed to implementation of this research.
Conflict of Interest
The authors declare that there are no conflicts of interest regarding the publication of this study. All stakeholders involved in the study, including pathology, laboratory quality, and nursing team members, worked collaboratively without competing financial or personal interests influencing the outcomes.
Data Availability
The data supporting this study’s findings, including incident reports, RAM evaluations, and audit results, are available upon reasonable request. Data access will be granted per institutional policies to ensure compliance with privacy regulations and ethical standards. Any request for data should be directed to the corresponding author, subject to approval from the hospital’s research ethics committee.
References
- Reducing sample mix-ups in molecular pathology: A review of current practices and future directions Smith R, Jones T. J Mol Diagn.2021;23(4):567-575.
- Short tandem repeat analysis: a practical tool to identify specimen mix-ups in the pathology laboratory João D, Cardoso S, Monteiro P, Leal C, Bartosch C. Virchows Archiv: An International Journal of Pathology.2023;483(4). CrossRef
- Quality assurance and quality control in molecular diagnostic laboratories. In: Practical Oncologic Molecular Pathology. Springer; 2021:77–85. Gao J GJ, Jennings LJ. KH. .
- Referral Process Enhancement: Innovative Approaches and Best Practices AlHarthy SH , Al-MoundhrI M, Al-Mahmoodi W, Ibrahim R, Ayaad O, Al Baimani K. Asian Pacific journal of cancer prevention: APJCP.2024;25(5). CrossRef
- Malpractice: An updated concept analysis and nursing implication in developing countries Al-Haijaa EA , Ayaad O, Al-Refaay M, Al-Refaay T. IOSR J Nurs Health Sci.2018;7(1):81-85.
- Reducing the Risk of Fall among Oncology Patients using Failure Modes and Effects Analysis Majed M, Ayaad O, AlHasni NS , Ibrahim R, AlHarthy SH , Hassan KK , Al-Zadjali R, Al-Awaisi H, Al-Baimani K. Asian Pacific journal of cancer prevention: APJCP.2024;25(2). CrossRef
- Quality control measures in molecular pathology: Current trends and future perspectives Miller J, Thompson B. J Pathol Inform.2021;12(1):45-52.
- Enhancing sample integrity in molecular pathology through risk management Williams K, Taylor H. Pathol Res Pract.2021;217(5):153-160.
- The role of automation in reducing sample handling errors in molecular pathology Johnson M, Lee S. J Clin Lab Autom.2023;29(3):211-220.
- Springer. Managing a PCR contamination event in a molecular pathology laboratory Available from: https://link.springer.com/protocol/10.1007/978-1-0716-2950-5_2.2023.
- Addressing sample mix-ups in oncology centers: A comprehensive review Garcia M, Hernandez F. Oncol Res Treat.2023;46(2):89-98.
- The role of total quality management in improving patient experiences and outcomes | Request PDF Haroun A, Ayaad O, Al-Ruzzieh MA , Ayyad M. ResearchGate.2024. CrossRef
- Improving diagnosis for patient safety in an oncology setting: Quality initiatives Al-Baimani K, Ayaad O, Ibrahim R, et al . Sobraj Publ Serv.2024.
- Examining Nurse and Patient Factors Before and After Implementing an Oncology Acuity Tool: A Mixed Methods Study Al-Ruzzieh MA , Eddin R, Ayaad O, Kharabsheh M, Al-Abdallah D. Journal of Nursing Measurement.2024;32(1). CrossRef
- Improving Care Continuity in Oncology Settings: A Lean Management Approach to Minimize Discharges Without Follow-Up Appointments AlHarthy SH , Ayaad O, Al Mashari AAA , AlBalushi MA , Ibrahim R, Bait Nasib MH , Al Zadjali R, Awaisi H, Al Baimani K. Asian Pacific journal of cancer prevention: APJCP.2024;25(4). CrossRef
- Implementing risk assessment matrices in clinical laboratories: A case study Brown L, Green [. Clin Lab Manag Rev.2022;34(2):123-130.
- The implications of artificial intelligence in the quality of health services Ayaad O. Innov Multidiscip J Sci Technol.2024;1(1):1-9.
- Organisational citizenship behaviour in the healthcare workplace: A scoping review Al-Ruzzieh MA , Ayaad O, Qaddumi B. Br J Healthc Manag.2022;28(6):1-7.
- Harnessing artificial intelligence in business: A qualitative exploration of strategic implementation and organizational impact Qaddumi B, Alshoaibi M, Alkhazaleh D, Ayaad O. Innov Multidiscip J Sci Technol.2024;1(1):10-17.
- The role of e-health in improving control and management of COVID 19 outbreak: current perspectives Al-Ruzzieh MA , Ayaad O, Qaddumi B. International Journal of Adolescent Medicine and Health.2020;34(4). CrossRef
- Measuring Occupational Fatigue among Higher and Middle Management at a Specialized Cancer Center during the COVID-19 Pandemic Al-Ruzzieh MA , Ayaad O. Asian Pacific journal of cancer prevention: APJCP.2022;23(10). CrossRef
- Risk assessment and mitigation strategies in molecular diagnostics Davis A, Patel R. Journal of Clinical Pathology.2022;75(7):456-462.
- Guidance for laboratories performing molecular pathology for cancer patients Cree IA , Deans Z, Ligtenberg MJL , Normanno N, Edsjö A, Rouleau E, Solé F, et al . Journal of Clinical Pathology.2014;67(11). CrossRef
License

This work is licensed under a Creative Commons Attribution-NonCommercial 4.0 International License.
Copyright
© Asian Pacific Journal of Cancer Biology , 2025
Author Details