Enhanced Anticancer Potential of Curcumin-Loaded Liposomal Nanoparticles in Oral Cancer Treatment
Download
Abstract
Objective: Curcumin, a naturally occurring polyphenol derived from turmeric, has demonstrated notable anticancer properties in various malignancies, including oral squamous cell carcinoma. However, its clinical application has been hampered by poor aqueous solubility and limited bioavailability. To address these challenges, we developed curcumin-loaded liposomal nanoparticles aimed at improving drug stability, enhancing solubility, and increasing cellular uptake in oral cancer cells.
Methods: Liposomal nanoparticles were prepared using the thin-film hydration method, followed by characterization of particle size, zeta potential, and drug encapsulation efficiency. The anticancer potential of free curcumin versus liposomal curcumin was evaluated in oral cancer cell lines (e.g., SCC-9 and HN-5) through cell viability and cytotoxicity assays (3-(4,5-dimethylthiazol-2-yl)-2,5-diphenyltetrazolium bromide, MTT). For in vitro drug release testing, the liposomal formulation containing curcumin was placed in a dialysis bag (molecular weight cutoff ~14 kDa) and immersed in phosphate-buffered saline (PBS) under continuous stirring at 37°C.
Results: Characterization revealed an average particle size of approximately 220 nm, a zeta potential of around -28 mV, and an encapsulation efficiency of about 82%. Compared to free curcumin, curcumin-loaded liposomes exhibited significantly enhanced antiproliferative effects in oral cancer cells, reflected by a 20% to 30% greater reduction in cell viability. Furthermore, the IC50 value for liposomal curcumin was substantially lower, highlighting its superior potency. In vitro drug release studies demonstrated a sustained release profile, with a moderate burst release observed in the initial hours, followed by a gradual release over 48 hours.
Conclusion: Curcumin-loaded liposomal nanoparticles offer a promising strategy to overcome the limitations of free curcumin by increasing its aqueous solubility, stability, and targeted delivery to cancer cells. The improved therapeutic efficacy and reduced off-target toxicity underscore the potential of this nanocarrier system as an effective treatment modality for oral cancer.
Introduction
Oral cancer often appears as squamous cell carcinoma in the mouth and remains a serious global health issue [1, 2]. Although treatments like surgery, radiotherapy, and chemotherapy have improved, they still cannot always fully control the tumor or maintain patients’ quality of life [3, 4]. As a result, researchers have turned their attention to natural compounds with anticancer effects, which generally have fewer side effects [4, 5]. Curcumin found in turmeric (Curcuma longa) is one of these promising compounds because of its anticancer, anti-inflammatory, and antioxidant properties [4, 5]. It can help block cancer progression in various cancers, including oral cancer [3, 6, 7]. Despite its potential, curcumin’s poor water solubility and low bioavailability limit its clinical use [4, 5, 8]. Large doses are often needed to achieve therapeutic levels, but these high doses can be expensive and toxic to healthy tissues [9, 10]. To overcome these problems, scientists are looking for new methods to boost curcumin’s effectiveness [3, 11]. Recently, nanotechnology and advanced drug delivery systems have gained attention for improving the performance of anticancer treatments and reducing side effects [9-12]. Liposomes widely considered safe and commonly used have a lipid bilayer structure similar to cell membranes, so they can carry both water-loving (hydrophilic) and water-hating (hydrophobic) substances at the same time [13, 14]. When curcumin is encapsulated in liposomes, it dissolves much better in watery solutions and stays stable longer inside the body [13, 15, 16]. Liposomes also help cancer cells take up more curcumin, improving the drug’s ability to slow cell growth and induce apoptosis in oral cancer cells [13, 17]. Putting curcumin in a liposomal delivery system offers several benefits. These include (1) boosting the amount of drug at the tumor site, (2) preventing the drug from breaking down too soon in the bloodstream, (3) reducing side effects on healthy tissue, and (4) releasing the drug slowly in a controlled way [9, 13, 14, 15, 16-19]. Overall, a nanoliposomal formulation of curcumin not only enhances its anticancer impact on oral cancer cells but also improves its pharmacological profile [9, 13]. The primary objective of this research is to design and evaluate a curcumin-loaded nanoliposomal formulation to enhance the solubility, stability, and cellular uptake of this compound in oral cancer cell lines. Accordingly, we plan to determine the formulation’s physicochemical properties, as well as assess its anticancer effects on oral squamous cell carcinoma cells. Through this effort, we aim to develop a more effective and less toxic therapeutic approach for oral cancer.
Materials and Methods
Materials
All essential reagents Curcumin, Cholesterol, Polyethylene Glycol 200, RPMI 1640 medium, Ethanol, Isopropanol, and Diethyl Ether were carefully sourced from Sigma to maintain high quality standards. Lecithin was obtained from Acros (Geel, Belgium). The SCC-9 and HN-5 oral cancer cell lines were generously provided by the Cell Bank of the Pasteur Institute of Iran.
Nanoliposomal Drug Preparation
Liposomal nanoparticles were fabricated using the reverse phase evaporation method, renowned for its high encapsulation efficiency of therapeutic payloads. Initially, a precisely quantified mixture of lecithin (14 mM), cholesterol (10 mM), PEG 200 (2 mM), and curcumin (2 mM) was solubilized in a combined ethanol/methanol phase to ensure uniform dispersion of all constituents. Under vacuum, this solution was subjected to rotary evaporation (120 rpm, 45°C), yielding a continuous, homogeneous lipid film. Subsequently, the resultant film was rehydrated with phosphate-buffered saline (PBS, pH 7.2), facilitating the spontaneous assembly of curcumin-entrapped liposomal vesicles. To refine particle size distribution and bolster colloidal stability, the nanoliposomal suspension underwent ten minutes of bath sonication at 50 W (Bandelin Sonopuls HD 2070, Bandelin Elec., Germany). Blank liposomes, prepared identically but devoid of the therapeutic agent, were concurrently synthesized to serve as experimental controls. To ascertain the mean diameter of the nanoliposome, a specially prepared sample was generated at a 1:25 ratio of nanoliposome to phosphate-buffered saline (pH 7.2). Nanoparticle concentration was quantified via absorbance measurements at 633 nm, while the hydrodynamic diameter and surface charge (zeta potential) were characterized using a Malvern Nano ZS3600 zetasizer. To determine the curcumin content within the formulations, atomic absorption spectroscopy (PerkinElmer, USA) was employed. The liposomal suspensions were subjected to high-speed centrifugation at 4°C and 12,000 rpm for 60 minutes, thereby facilitating the partitioning of encapsulated versus free drug. Following each centrifugation cycle, the supernatant was meticulously aspirated and discarded, leaving the liposomal pellet. Encapsulation efficiency (EE%) was subsequently calculated by subtracting the concentration of free curcumin (remaining in the supernatant) from the total initial curcumin content, as expressed by the following equation:
{EE%} = {Total Anti-Cancer Drug} - {Free Anti- Cancer Drug} / {Total Anti-Cancer Drug} × 100.
In vitro Drug Release Study
A rigorous assessment of carrier-mediated drug release kinetics was conducted via a membrane diffusion assay in a phosphate-buffered saline medium (PBS, pH 7.2). In this protocol, a precisely measured 2 mL aliquot of the drug-loaded formulation encompassing 0.12 mg of the active compound was enclosed in dialysis bags (cellulose membrane, 14 kDa cutoff). These sealed bags were then submerged in 120 mL of PBS, maintained at 37°C, and continuously stirred at 120 rpm to ensure a uniform diffusion gradient. At predetermined intervals (1, 5, 8, 14, 24, 34, and 48 hours), aliquots were carefully withdrawn from the external PBS reservoir and replaced with fresh buffer to preserve both total volume and concentration gradient. The withdrawn samples were subsequently analyzed using high-performance liquid chromatography (HPLC, Shimadzu, Japan) a technique renowned for its high precision to quantify the amount of drug diffused over time. The resultant release profiles were benchmarked against those of unencapsulated drug samples by employing a rigorously generated standard curve, thus providing a comprehensive evaluation of the carrier system’s efficacy in modulating drug discharge.
MTT test
The cytotoxic efficacy of the curcumin-based formulation was determined via the MTT assay and benchmarked against the conventional reference drug. Specifically, SCC-9 and HN-5 cell lines were seeded into 96-well plates and allowed to proliferate for 24 hours prior to exposure to varying concentrations of either the formulated curcumin or its free-drug counterpart for 48 hours. Subsequently, the MTT solution was introduced, followed by a one-hour incubation to enable formazan crystal formation. The supernatant was then discarded and replaced with isopropanol to solubilize the crystals, after which the absorbance at 570 nm was recorded using an ELISA microplate reader. The percentage of cytotoxicity was derived according to the following relationship:
Cytotoxicity (%) = [1 − (Absorbance of treated cells / Absorbance of control)] × 100
Cell viability was then calculated by subtracting the cytotoxicity value from 100%. The half-maximal inhibitory concentration (IC₅₀) was subsequently ascertained via the Pharm software.
Statistical Analysis
All data underwent comprehensive 1statistical evaluation using SPSS (version 11), while every phase of toxicity assessment was conducted through the Pharm software platform.
Results
Characterization of nanoparticles
Characterization data indicated that the nanoformulation possessed an average particle size of 220 ± 6 nm (as determined by dynamic light scattering), accompanied by a zeta potential of approximately −28 ± 2 mV. These findings suggest a relatively stable colloidal system with limited agglomeration tendencies. Additionally, the encapsulation efficiency was estimated at 82 ± 3%, reflecting a notably high capacity for incorporating the active compound within the nanoparticulate structure (Table 1).
Parameter | Value | Remarks |
Average Particle Size | 220 ± 6nm | Determined via dynamic light scattering (DLS) |
Zeta Potential | −28 ± 2mV | Indicates colloidal stability and reduced aggregation |
Encapsulation Efficiency | 82 ± 3% | Demonstrates high active-compound loading capacity |
Drug release study
A comprehensive examination of curcumin release kinetics from nanoparticulate systems was conducted via the membrane diffusion approach in phosphate-buffered saline (PBS) at a pH of 7.2. Intriguingly, the resultant release profiles exhibited a pronounced biphasic behavior, typified by an early burst phase followed by a gradual, sustained discharge over the ensuing 48 hours. During the meticulous dialysis procedure at the designated pH, the encapsulated curcumin maintained a measured and predictable release trajectory. Notably, quantitative analysis revealed that approximately 58% of the encapsulated curcumin was liberated from the liposomal constructs by the conclusion of the 48-hour observation window, as depicted in Figure 1.
Figure 1. In Vitro Drug Release Studies Demonstrated a Sustained Release Profile, with a Moderate Burst Release in the Initial Hours, Followed by a Gradual Release Over 48hours (n=3). Error bars represent the standard error of the mean (SEM).
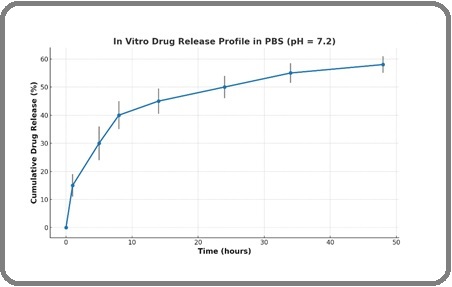
In vitro cytotoxicity assay
The cytotoxic efficacy of curcumin-loaded liposomes was systematically assessed in comparison to that of conventional (free) curcumin using SCC-9 and HN-5 oral cancer cell lines. Both cell types were exposed to equivalent concentrations of the liposomal formulation and the free drug for a 48-hour incubation period at 37°C. As depicted in Figure 2, the resulting dose-response profiles reveal a marked enhancement in cytotoxic potency for the liposomal curcumin, as evidenced by significantly lower IC₅₀ values relative to its free counterpart.
Figure 2. Cytotoxic Comparison between Liposomal Curcumin and Free Curcumin in SCC-9 and HN-5 Cells after 48 hours of Treatment (n = 3, *: p < 0.05,). Error bars represent the standard error of the mean (SEM)..
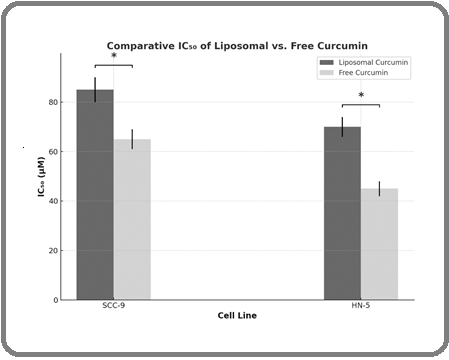
Specifically, in SCC-9 cells, the IC₅₀ values were 85 µM for the liposomal formulation and 65 µM for free curcumin, whereas in HN-5 cells, the corresponding values were 70 µM and 45 µM, respectively. These findings suggest that liposomal encapsulation substantially augments curcumin’s cytotoxic activity, likely attributable to improved bioavailability, enhanced intracellular uptake, and a sustained release profile. The superior anticancer efficacy exhibited by the liposomal curcumin highlights its promise as a more potent therapeutic modality for the treatment of oral malignancies (Figure 2).
Discussion
Oral cancer is one of the most prevalent malignancies within the head and neck region, often associated with poor prognosis, high recurrence rates, and resistance to conventional therapies [20]. Consequently, there is an urgent need for the development of targeted and effective therapeutic strategies with reduced systemic toxicity [21-22]. In this context, natural compounds with anticancer potential, such as curcumin, have garnered considerable attention [23]. To overcome these limitations, advanced nanostructured drug delivery systems including liposomes, polymeric nanoparticles, lipid-based carriers, and nanoemulsions have been developed [24-26].
These platforms enhance the solubility, stability, and bioavailability of curcumin, while enabling targeted and controlled drug release within tumor microenvironments [27]. Among them, liposomal curcumin formulations have shown particular promise due to their nanoscale size, biocompatibility, and improved cellular uptake [28]. The physicochemical characterization of the curcumin-loaded liposomal formulation revealed a uniform average particle size of approximately 220 ± 6 nm, which falls within the optimal nanoscale range for efficient cellular uptake and enhanced permeability across biological membranes. The negative zeta potential (−28±2mV) suggests strong colloidal stability and minimized particle aggregation, thereby contributing to prolonged circulation and predictable behavior under physiological conditions [29]. Furthermore, the high encapsulation efficiency (82 ± 3%) underscores the formulation’s capacity to effectively incorporate curcumin within the liposomal bilayers, thereby enhancing its solubility and protecting it from premature degradation [30]. In vitro drug release analysis demonstrated a distinct biphasic release profile in phosphate-buffered saline (pH 7.2), characterized by an initial burst release followed by a sustained release over 48 hours. This kinetic behavior is particularly advantageous in therapeutic contexts, as the early burst may facilitate rapid attainment of therapeutic drug levels, while the subsequent controlled release phase ensures prolonged exposure of cancer cells to the bioactive compound [31]. The release data, showing approximately 58% cumulative release at 48 hours, further validates the formulation’s ability to modulate drug delivery over time an essential criterion for enhancing bioavailability and therapeutic efficacy. The cytotoxicity profiles observed in SCC-9 and HN-5 oral cancer cell lines substantiate the functional advantages of liposomal encapsulation. Compared to free curcumin, the liposomal formulation exhibited significantly lower IC₅₀ values in both cell lines, indicating enhanced antiproliferative activity. The greater cytotoxic potency of liposomal curcumin can be attributed to improved cellular uptake mechanisms associated with nanocarrier systems, as well as sustained intracellular release, which collectively facilitate increased drug accumulation within cancer cells. The disparity in IC₅₀ values between liposomal and free forms particularly in HN-5 cells (70 µM vs. 45 µM) further emphasizes the formulation’s potential for selective and enhanced targeting of malignant cells.
In conclusion, technology has greatly contributed to the advancement of various malignancies and other fields [32-39]. Technology has emerged as a key driver of progress across various scientific and industrial fields. In recent years, advanced technological tools have significantly improved the diagnosis and treatment of numerous diseases, including cancer [40]. Moreover, novel processing techniques such as plasma and dielectric barrier discharge have enabled the fabrication of porous, customizable scaffolds with enhanced mechanical properties for diverse applications [41]. In the realm of personalized medicine, next-generation sequencing technologies have played a crucial role in tailoring treatment approaches for complex diseases, leading to more effective patient-specific interventions [42]. Advanced materials engineering has also benefited from these technological developments, resulting in composite materials with tailored crystallinity and improved mechanical performance [43]. Furthermore, the application of innovative stochastic optimization models and modern management strategies has enhanced the resilience and efficiency of pharmaceutical supply chains, particularly under the pressures of global health crises [44-45]. In addition, artificial intelligence-based decision-making tools have been effectively applied to improve criteria ranking in sectors such as health tourism, demonstrating the broad applicability of these technologies [46]. Recent studies highlight the growing expansion of advanced technologies such as deep learning, neural networks, and experimental innovations across various fields including biomedical research, renewable energy, and hydrological forecasting, clearly demonstrating the transformative role of these technologies in addressing complex scientific and engineering challenges [47-52]. These findings support the hypothesis that liposomal delivery systems not only improve the physicochemical stability and bioavailability of hydrophobic compounds like curcumin but also amplify their anticancer efficacy. The integration of favorable nano-scale characteristics with controlled drug release and enhanced cytotoxic response positions this liposomal formulation as a promising candidate for further development in oral cancer therapeutics.
Acknowledgements
None.
Data availability
Not applicable as we used information from previously published articles.
Approved by any scientific Body
Not applicable as the manuscript is not a part of any student thesis.
Consent for publication
All authors have given consent for publication.
Conflict of interest
The authors declare no potential conflict of interest.
References
- The prevalence of squamous cell carcinoma in different sites of oral cavity at our Rural Health Care Centre in Loni, Maharashtra - a retrospective 10-year study Tandon P, Dadhich A, Saluja H, Bawane S, Sachdeva S. Contemporary Oncology (Poznan, Poland).2017;21(2). CrossRef
- Squamous Cell Carcinoma of the Oral Cavity: A Case Series Analysis of Clinico-Pathological Features Saini N, Manjunatha B, Shah V, Pateel D. International Journal of Oral and Maxillofacial Pathology.;10.
- In vitro and in vivo effects of curcumin on oral cancer: a systematic review Ridho F, Syachputra A, Fahrudin P, Nurhuda A, Nurliana N, Latuamury N. Current Biomedicine.. CrossRef
- Therapeutic potential of curcumin and its nanoformulations for treating oral cancer Mukherjee D, Krishnan A. World Journal of Methodology.2023;13(3). CrossRef
- Role of curcumin in oral cancer prevention Suhail S, Gupta S, Kumar V, Gupta O. ResearchGate.2024. CrossRef
- Curcumin-induced autophagy contributes to the decreased survival of oral cancer cells Kim JY , Cho TJ , Woo BH , Choi KU , Lee CH , Ryu MH , Park HR . Archives of Oral Biology.2012;57(8). CrossRef
- Curcumin inhibits oral squamous cell carcinoma SCC-9 cells proliferation by regulating miR-9 expression Xiao C, Wang L, Zhu L, Zhang C, Zhou J. Biochemical and Biophysical Research Communications.2014;454(4). CrossRef
- Curcumin: New Weapon against Cancer Hamam F. Food and Nutrition Sciences.2014;5(22). CrossRef
- Use of Liposomal Curcumin for Oral Cancer Treatment: A Systematic Review and Meta Analysis Bhagat N, Salam S, Garg A, Venkatesan V, Hardik S, Hardik SG , Kumar S, Kashwani R. Nanotechnology Perceptions.2024. CrossRef
- Curcumin combined with photodynamic therapy, promising therapies for the treatment of cancer Xie L, Ji X, Zhang Q, Wei Y. Biomedicine & Pharmacotherapy = Biomedecine & Pharmacotherapie.2022;146. CrossRef
- Curcumin-Encapsulated Nanomicelles Improve Cellular Uptake and Cytotoxicity in Cisplatin-Resistant Human Oral Cancer Cells Kumbar VM , Muddapur U, Bin Muhsinah A, Alshehri SA , Alshahrani MM , Almazni IA , Kugaji MS , et al . Journal of Functional Biomaterials.2022;13(4). CrossRef
- Nanoliposomal Coencapsulation of Dorema aucheri Extract and Curcumin; Enhanced Cytotoxicity, Apoptosis Induction, and Inhibition of EGFR Gene Expression in Oral Cancer Cells OCC-02 Azizi M, Ghalamfarsa G, Khosravani F, Bardania H, Azizi S. IET nanobiotechnology.2023;2023. CrossRef
- Liposomal curcumin and its application in cancer Feng T, Wei Y, Lee RJ , Zhao L. International Journal of Nanomedicine.2017;12. CrossRef
- Silica-coated flexible liposomes as a nanohybrid delivery system for enhanced oral bioavailability of curcumin Li C, Zhang Y, Su T, Feng L, Long Y, Chen Z. International Journal of Nanomedicine.2012;7. CrossRef
- Enhancing stability and anti-inflammatory properties of curcumin in ulcerative colitis therapy using liposomes mediated colon-specific drug delivery system Wang C, Han Z, Wu Y, Lu X, Tang X, Xiao J, Li N. Food and Chemical Toxicology: An International Journal Published for the British Industrial Biological Research Association.2021;151. CrossRef
- Liposomes coated with thiolated chitosan as drug carriers of curcumin Li R, Deng L, Cai Z, Zhang S, Wang K, Li L, Ding S, Zhou C. Materials Science & Engineering. C, Materials for Biological Applications.2017;80. CrossRef
- 23 Liposomal Curcumin Reduces Growth In Head And Neck Squamous Cell Carcinoma Cell Lines Tang C, Ho B, Wang D, Veena M, Ahmed S, Srivatsan E, Wang M. Journal of Investigative Medicine.2007;55. CrossRef
- Abstract 4227: Curcumin in cyclodextrin in liposome as a delivery vehicle against osteosarcoma Dhule S, Penfornis P, Frazier T, Walker R, Tan G, He J, John V, Pochampally R. Cancer Research.2024;71:4227. CrossRef
- Inducing sustained release and improving oral bioavailability of curcumin via chitosan derivatives-coated liposomes Tian M, Song R, Wang T, Sun M, Liu Y, Chen X. International Journal of Biological Macromolecules.2018;120(Pt A). CrossRef
- Chitosan Encapsulated Curcumin Nanoparticles As An Effective Drug Delivery System For Oral Cancer Treatment Keerthikumar, Jalalpure S, Rao P. Indian Drugs.2015. CrossRef
- Prospects of Curcumin Nanoformulations in Cancer Management Amekyeh H, Alkhader E, Sabra R, Billa N. Molecules (Basel, Switzerland).2022;27(2). CrossRef
- Pharmacokinetics of Curcumin Delivered by Nanoparticles and the Relationship with Antitumor Efficacy: A Systematic Review Silvestre F, Santos C, Silva V, Ombredane A, Pinheiro W, Andrade L, Garcia M, et al . Pharmaceuticals (Basel, Switzerland).2023;16(7). CrossRef
- Curcumin nanoformulations: Beneficial nanomedicine against cancer Maleki Dizaj S, Alipour M, Dalir Abdolahinia E, Ahmadian E, Eftekhari A, Forouhandeh H, Rahbar Saadat Y, Sharifi S, Zununi Vahed S. Phytotherapy research: PTR.2022;36(3). CrossRef
- Advances in nanotechnology-based delivery systems for curcumin Sun M, Su X, Ding B, He X, Liu X, Yu A, Lou H, Zhai G. Nanomedicine (London, England).2012;7(7). CrossRef
- Pharmacokinetic Study of Nanoparticulate Curcumin: Oral Formulation for Enhanced Bioavailability Ravichandran R. Journal of Biomaterials and Nanobiotechnology.2013;4. CrossRef
- Curcumin delivery and co-delivery based on nanomaterials as an effective approach for cancer therapy Pourmadadi M, Abbasi P, Eshaghi MM , Bakhshi A, Ezra Manicum A, Rahdar A, Pandey S, et al . Journal of Drug Delivery Science and Technology.2022;78. CrossRef
- Surface modification of solid lipid nanoparticles for oral delivery of curcumin: Improvement of bioavailability through enhanced cellular uptake, and lymphatic uptake Baek J, Cho C. European Journal of Pharmaceutics and Biopharmaceutics: Official Journal of Arbeitsgemeinschaft Fur Pharmazeutische Verfahrenstechnik e.V.2017;117. CrossRef
- Synthesis and characterization of acetyl curcumin-loaded core/shell liposome nanoparticles via an electrospray process for drug delivery, and theranostic applications Reddy AS , Lakshmi BA , Kim S, Kim J. European Journal of Pharmaceutics and Biopharmaceutics: Official Journal of Arbeitsgemeinschaft Fur Pharmazeutische Verfahrenstechnik e.V.2019;142. CrossRef
- Assessing the potential of liposomes loaded with curcumin as a therapeutic intervention in asthma Ng ZY , Wong J, Panneerselvam J, Madheswaran T, Kumar P, Pillay V, Hsu A, et al . Colloids and Surfaces. B, Biointerfaces.2018;172. CrossRef
- Studying the Characteristics of Curcumin-Loaded Liposomal Nanoparticles Afyouni I, Ghanbarikondori P, Pour N, Hashemian P, Jalali F, Sedighi A, Allahyartorkaman M. ResearchGate.. CrossRef
- Injectable and In Situ-Formable Thiolated Chitosan-Coated Liposomal Hydrogels as Curcumin Carriers for Prevention of In Vivo Breast Cancer Recurrence Li R, Lin Z, Zhang Q, Zhang Y, Liu Y, Lyu Y, Li X, et al . ACS Applied Materials & Interfaces.2020;12(15). CrossRef
- Introduction of a New Nano Sized Ni-Based Salt for the Acceleration of the Synthesis of Pyrano[2,3-d]pyrimidinone and 1,4-Dihydropyridine Derivatives Yarinia R, Shirini F, Langarudi MSN , Seyyedi N. Polycyclic Aromatic Compounds.2022;42(10). CrossRef
- Inflammatory reflex disruption in COVID-19 Hajiasgharzadeh K, Jafarlou M, Mansoori B, Dastmalchi N, Baradaran B, Khabbazi A. Clinical and Experimental Neuroimmunology.2022;13(4). CrossRef
- The modulatory role of dendritic cell-T cell cross-talk in breast cancer: Challenges and prospects Shahverdi M, Masoumi J, Ghorbaninezhad F, Shajari N, Hajizadeh F, Hassanian H, Alizadeh N, Jafarlou M, Baradaran B. Advances in Medical Sciences.2022;67(2). CrossRef
- Dual silencing of tumor-intrinsic VISTA and CTLA-4 stimulates T-cell mediated immune responses and inhibits MCF7 breast cancer development Hosseinkhani N, Hemmat N, Baghbani E, Baghbanzadeh A, Kazemi T, Mokhtarzadeh A, Jafarlou M, Amin Doustvandi M, Baradaran B. Gene.2024;896. CrossRef
- The Combination of PD-L1 and CTLA-4 Suppression Significantly Decreased the Expression Levels of Cancer Stem Cell Factors in the Pancreatic Cancer Cell Line Alizadeh, Nazila , Tohid Kazemi , Nima Hemmat , Mahdi Jafarlou , Behzad Baradaran . ImmunoAnalysis.2024;3(1):6. CrossRef
- Combination of B7H6-siRNA and temozolomide synergistically reduces stemness and migration properties of glioblastoma cancer cells Allahyarzadeh Khiabani N, Amin Doustvandi M, Mohammadnejad F, Salmani Hassan Kohal E, Boushehri N, Jafarlou M, Baradaran B. Experimental Cell Research.2023;429(1). CrossRef
- Unveiling the menace: a thorough review of potential pandemic fungal disease Jafarlou M. Frontiers in Fungal Biology.2024;5. CrossRef
- siRNA-mediated inhibition of survivin gene enhances the anti-cancer effect of etoposide in U-937 acute myeloid leukemia cells Jafarlou M., Baradaran B., Shanehbandi D., Saedi T. A., Jafarlou V., Ismail P., Othman F.. Cellular and Molecular Biology (Noisy-Le-Grand, France).2016;62(6). CrossRef
- Downregulation of LPAR1 Promotes Invasive Behavior in Papillary Thyroid Carcinoma Cells Bokaii Hosseini Z, Rajabi F, Morovatshoar R, Ashrafpour M, Behboodi P, Zareie D, Natami M. Cancer Informatics.2024;23. CrossRef
- Polymerization of Sodium 4-Styrenesulfonate Inside Filter Paper via Dielectric Barrier Discharge Plasma Amiri Khoshkar Vandani S, et al . Plasma.2024;7(4):867-876. CrossRef
- The Role of Next Generation Sequencing Panels in Personalized Medicine of Lung Cancer: A Review Study Pezeshki M, Zangenehnejad Z, Tamjidi E, Beig Verdi H, Nakhaee M, Ziaei S, Maddahian-Najafabadi P, et al . Personalized Medicine Journal.2024;9(35). CrossRef
- Sulfur-driven reactive processing of multiscale graphene/carbon fiber- polyether ether ketone (PEEK) composites with tailored crystallinity and enhanced mechanical performance Motta de Castro E, Bozorgmehrian F, Carrola M, Koerner H, Samouei H, Asadi A. Composites Part B: Engineering.2025;295. CrossRef
- A Multistage Stochastic Optimization Model for Resilient Pharmaceutical Supply Chain in COVID-19 Pandemic Based on Patient Group Priority Mahdavimanshadi M, et al . IEEE.2024 SIEDS; 2024;:382-387. CrossRef
- Green Drug Supply Chain Investigation by Time-Market Balance and Risk Entezami M, Havaeji H. World Journal of Engineering and Technology.2023;11(3):611-631. CrossRef
- Ranking of AI-Based Criteria in Health Tourism Using Fuzzy SWARA Method Basirat S, et al . Computer and Decision Making: An International Journal.2025;2:530-545. CrossRef
- Berberis vulgaris fruit crude extract as a novel anti-leukaemic agent Saedi T. A., Ghafourian S., Jafarlou M., Sabariah M. N., Ismail P., Eusni R. M. T., Othman F.. Journal of Biological Regulators and Homeostatic Agents.2015;29(2).
- Improving Long-Term Flood Forecasting Accuracy Using Ensemble Deep Learning Models and Attention Mechanism Kordani M, Nikoo MR , Fooladi M, Ahmadianfar I, Nazari R, Gandomi AH . ResearchGate.2025. CrossRef
- Optimized classification of dental implants using convolutional neural networks and pre-trained models with preprocessed data Lashaki RA , Raeisi Z, Razavi N, Goodarzi M, Najafzadeh H. BMC Oral Health.2025;25(1). CrossRef
- Multi-Step Ahead Water Level Forecasting Using Deep Neural Networks Sharafkhani, Fahimeh , Steven Corns , Robert Holmes . Water.2024;16(21):3153. CrossRef
- Dendrite neural network scheme for estimating output power and efficiency for a class of solar free-piston Stirling engine Lashaki RA , Raeisi Z, Makki M, Zare S. International Journal of Modelling and Simulation.;0(0). CrossRef
- Introducing a novel temperature measurement to analyze the effect of hybrid cooling methods on improving solar panel performance: An experimental approach Aali M, Mansouri M, Raeisi Z, Lashaki RA , Tavakoli S. Applied Thermal Engineering.2025;268. CrossRef
License

This work is licensed under a Creative Commons Attribution-NonCommercial 4.0 International License.
Copyright
© Asian Pacific Journal of Cancer Biology , 2025
Author Details