Advances in Targeted Drug Delivery in Melanoma
Download
Abstract
Cancer remains a major killer of mankind. Failure of conventional chemotherapy has resulted in recurrence and development of virulent multi drug resitant (MDR) phenotypes adding to the complexity and diversity of this deadly disease. Melanoma is the most aggressive and dangerous type of skin cancer, but its molecular mechanisms remain largely unclear Drug delivery systems (DDS) such as lipid- or polymer-based nanoparticles can be designed to improve the pharmacological and therapeutic properties of drugs administered parenterally with the emergence of nanotechnology, the use of nano-carriers is widely expected to alter the landscape of melanoma treatment multifunctional nanoparticles that can integrate various key components such as drugs, genes, imaging agents and targeting ligands using unique delivery platforms would be more efficient in treating cancers. This review presents some of the important principles involved in development and novel methods of treating cancers using multifunctional-targeted nanopicles. Illustrative examples of nanoparticles engineered for drug/gene combination delivery and stimuli respnsive nanoparticle systems for cancer therapy are also discussed.
Introduction
In the world, cancer remains one of the leading causes of the death of many in this world. According to the recent report of the International Agency for Research on Cancer reported that around a 19 million new cases of cancer each year and the death of 9 million for the year 2020 [1]. Melanoma is a offenly seen in people is quite frequent. Melanoma incidence is not associated with age. Most cancer deaths were caused by people of age around 20-35. Melanoma genesis is the means of acquiring successive changes for certain transcription factors that regulate biochemical as well as cellular factors that regulate critical cell activities. It also helps us to understand the cooperation of genetics and the environment in nature [2]. The early detection of cancer can be curable in many ways such as medical and surgical removal of damaged organs or cells. cancer cells migrating from their primary site to another portion of the body. Cancer cells detach away from the initial (primary) tumor, move via the circulatory or lymphatic system, and form a new nodule in other parts or tissues within the body in a process known as metastasis. Early diagnosis of melanoma is critical since individuals without the advanced disease have a 90% five-year survival rate. Because metastatic melanoma is noted for its high drug resistance, therapeutic possibilities for individuals in this stage are substantially more constrained. Early identification of melanoma is limited by the lack of adequate tumor markers and public education, as well as the lack of clinically significant symptoms until the illness had advanced [3]. Surgery, radiation therapy, chemotherapy, immunotherapy, and targeted therapy are now the five main areas of standard therapies for melanoma patients. The tumor as well as some surrounding healthy tissue are removed during surgery, depending on the stage of cancer and the individual’s risk of recurrence. Wide excision, lymphatic mapping and sentinel lymph node biopsy, and lymph node dissection are among therapies used to treat regional and local metastasis [4]. Carcinoma progression is a multistage process with clinical and histological features [5]. Melanoma genesis may be split into five phases histologically. Acquired spots develop in stage one as a result of enhanced melanocyte proliferation [6]. Although cells are low - grade squamous intraepithelial lesions, they are the source of the majority of malignant melanomas.
Stages of melanoma
Stage 0: The cancer is confined to the epidermis region, the outermost skin layer. It has not spread to nearby lymph nodes or distant parts of the body.
Stage 1: tumor with the size of less than two mm thick, it may be or may not be ulcerated. Cancer has not spread to nearby lymph nodes or distant parts of the body.
Stage II: The tumor is more than 1 mm thick and maybe thicker than 4 mm. it may be or may not be tumor growth. Stage III A: The tumor is no more than 2 mm thick and might or might not be ulcerated. It may be spread nearby lymphatic vessels, but it is so small that it is only seen under the microscope.
Stage III B: There is no sign of the primary tumor: Cancer has spread nearby lymphatic vessels. It has spread to very small areas of nearby parts or lymphatic channels around the tumor and may not affect nearby cells or other body parts
Stage III C: The tumor is more than 2 mm but no more than 4 mm thick and is ulcerated it is thicker than 4 mm but is not ulcerated. Cancer has spread to nearby lymph nodes, which and have reached nearby lymph nodes.
Stage III D: The tumor is thicker than 4 mm and is ulcerated more nearby lymph nodes. tumor and may affect nearby cells or other body parts.
Stage IV: The tumor can be any thickness and might or might not be ulcerated. Cancer might or might not have spread to nearby lymph nodes. It has spread to distant lymph nodes and effect the other organs.
Along with chemo and surgery, radiation therapy is one of the most common cancer treatments. 50 percent of all cancer patients will be treated with RT, either alone or in combination with other therapies, in contemporary clinical cancer therapy [7]. Radioactive material is used during RT to destroy cancer cells and prevent tumor growth and recurrence. External beam radiation (EBRT) and internal radioisotope treatment are the two major categories of RT (RIT). Radiation beams from outside the body, such as high-energy x-rays, electron or proton beams, are directed directly onto tumors in EBRT to trigger cancer cell death. In the case of internal RIT, therapeutic radioactive isotopes would be delivered to the tumor cells through minimally invasive procedures such as direct infusion through a catheter known as brachytherapy or systemic administration of suitable tumor-homing carriers (such as antibodies, liposome emulsions, or nanoparticles with tumor-targeting ligands. While EBRT has been widely utilized to treat local solid tumors, RIT is used to treat both local and metastatic tumors that have traveled through the bloodstream. Radiation therapy has a variety of adverse effects, including dermatitis, allergies, and tiredness [8]. After brachytherapy, these symptoms generally improve within a few weeks. Radiation-induced skin responses can be prevented and treated using topical corticosteroid creams and antibiotics. Lymphedema is a long-term, persistent adverse effect of some medications [9].
Immunotherapy a type of cancer treatment that works by boosting the body’s natural defenses. Immunotherapy has made significant progress in treating stage III and stage IV melanoma in recent years. Although immunotherapy can be successful in the treatment of melanoma, there are a lot of potential adverse effects. Currently, the FDA has authorized two types of immunotherapy: (1) inhibitors of the programmed death receptor 1 (PD-1) or its ligand (PD-L1), and (2) cytotoxic T-cell lymphocyte-associated protein 4 (CTLA-4) (CTLA-4) [10]. In the study of new targeted therapies, proto-oncogene protein CTLA4 or CTLA-4 (cytotoxic T-lymphocyte-associated protein 4) is a protein receptor that functions as an immune checkpoint and downregulates immune responses. T cells (a kind of immune cell) contain a protein that helps regulate the body’s immunological responses. CTLA-4 helps prevent T cells from destroying other cells, particularly cancer cells when it is linked to another protein called B7. Despite progress in the analysis of metastatic melanoma, medication resistance and systemic toxicity pose a threat to effectiveness. In comparison to other cancer types, melanoma therapy has a poor overall success rate.
Researchers have created nanoparticle (NP) technology as a method of solving the difficulties of off-target effects, significant toxic side effects, and limited circulation period in traditional systemic drug delivery. Recent advancements in Nano-medicine have made it simpler for researchers to design successful therapies recent years. Immunomodulators can be used to downregulate oncogenes or reestablish the tumor suppressor environment for further efficient cancer treatment, as Nanoparticles provide a good protective barrier against host immune response assault and enzymatic disintegration [11]. Numerous scientific advances in melanocyte science, relevant oncogenic genetic abnormalities, chromosomal aberrations and point mutations in melanoma, the impact of molecular signaling pathways on melanomagenesis, and the interaction of aberrant signaling pathways with host immune elements have greatly expanded what is known over the last three decades. This newfound knowledge has resulted in significant advancements in the diagnosis, categorization, and therapy of this condition. This is a remarkable achievement that is becoming increasingly important as the number of new cases and fatalities from melanoma continues to grow.
Biology of Melanocytes
Melanoma cells are noted for having a high rate of mutation. Due to its great heterogeneity and propensity to escape the immune system and acquire multidrug resistance, melanoma is exceedingly difficult to treat, leaving subsequent treatments unsuccessful. Various melanoma risk factors have been identified in epidemiological research over the last few decades
The molecular processes of melanoma development might be extremely different. UV exposure, for example, stimulates keratinocytes to express more of the multifunctional protein p53 that, functioning as a transcriptional factor, leads these cells to produce more melanocyte-stimulating hormone (MSH) [12]. MSH protein secreted on the surface membrane of melanocytes attach to melanocortin 1 receptors (MC1R), which enhance the intracellular adenylate cyclase cascade and stimulate the CREB pathway, leading to the activation of transcriptional factor MITF [13]. MITF subsequently transactivates the expression of p16 and Bcl2 protein, which support melanocytes’ survival [14]. Invasive and metastatic melanocytes can also inhibit the immune system function, for example, by increasing the production of CTLA-4 protein receptors, which inhibit the activity of T-cells [15]. Ipilimumab, a newly discovered anticancer medication that targets this protein, has been shown to improve survival in late melanoma patients [16].
Oncogenic Pathways
The first monoclonal antibodies against CTLA-4 enabled the first direct studies of its activation and a better understanding of its activity [17]. The threshold model, in which CTLA-4 sets a threshold of activation above the background “noise” of T- cell receptor signals, and the attenuation model, in which CTLA4 limits the capacity of a T cell to divide after initiation of activation [18] have both been proposed for CTLA-4 regulation of T-cell expansion. CTLA-4 is regarded as an immunological checkpoint blockade because it inhibits potential autoreactive T cells at the early stages of naïve T-cell activation, often in lymphatic vessels [19]. CTLA-4 is not expressed by T cells at steady-state but is upregulated within 24–48 h after activation [20]. CTLA4 (CD152) contains a combination of intercellular adhesion that can stop initial processes within the receptor-mediated signaling pathway. It is a type 1 transmembrane protein of the immunoglobin family of proteins with 223 amino acid residues and a molecular weight of 41–43 kDa. A single IgV-like domain containing the B7-1 (CD80)/B7-2 (CD86) ligand-binding site defines CTLA-4’s extracellular architecture. TCR activation by antigens and CD28-B7 interaction are both required for CTLA4 expression. The CTLA4 co-stimulatory mechanism suppresses or down-regulates. CTLs (Cytotoxic T-Lymphocytes) are capable of killing these cells by triggering apoptosis [21]. CTLA4 is an important inhibitor of T-cell activation. It is not continuously released but is increased in response to TCR activation, with more activation resulting in more CTLA4 at the cell surface membrane [22]. T- Cell receptors binding to Major Histocompatabile specifies T-cell activation, but another co-stimulatory signaling is necessary. Adhesion of B7-1 (CD80) or B7-2 (CD86) proteins on the APC to CD28 molecules on the T cell results in a T cell signal. CTLA4 dimers form a linear zipper-like structure between B7-1/B7-2 and CTLA-4 dimers, but each CTLA4 dimer might attach two different B7-1/B7-2 homodimers [23]. PI3K (Phosphatidylinositol 3-Kinase), the tyrosine phosphatases SHP1 and SHP2, and the serine/threonine phosphatase PP2A are all activated by CTLA4. PP2A targets phosphoserine/threonine residues and is known to interfere with Akt activation. SHP1 and SHP2 dephosphorylate TCR-signaling proteins, whereas SHP1 and SHP2 target phosphoserine/threonine residues. CTLA4 binding to PI3K suggests that the co-receptor, like CD28, can provide positive signals (Figure 1).
Figure 1. T-cell Response and Tumor Cell Response Activation.
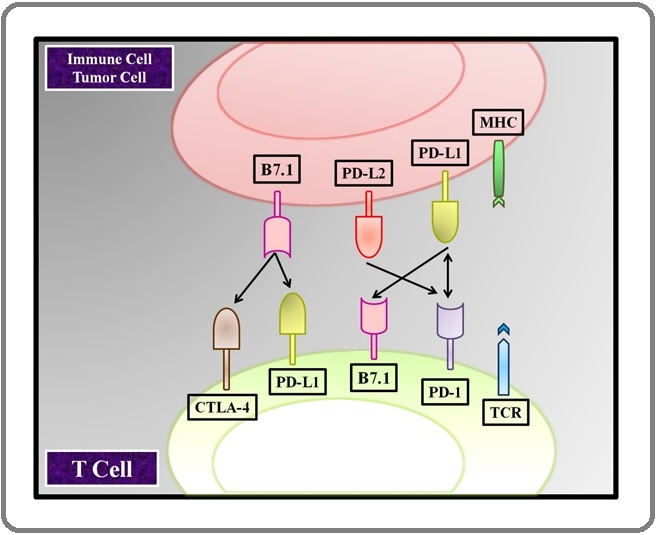
T cells, through the release of expansion factors like interleukin-2 (IL-2), enhanced energy consumption, and upregulation of cell survival genes enhanced T-cell viability and development. Because CTLA4 has a significantly greater affinity for B7 to CD28, one method includes antagonism of B7-CD28–mediated costimulatory signals by CTLA4. CTLA4 binds CD80/86 500–2500 times more effectively than CD28. CD28 activation increases IL-2 mRNA synthesis and cellular stage activation, T-cell viability, T-helper cell development, and immunoglobulin isotype switching. CTLA4 signaling prevents the synthesis of IL-2 mRNA and the development of the cell cycle. This process is solely dependent on CTLA4’s extracellular domain and operates in a proportionate manner to the degree of upregulation. CTLA-4 is influenced by a variety of factors, primarily subcellular level. CTLA-4 is predominantly found in the cytoplasmic membrane of resting naïve T lymphocytes. [14]. Receptor-mediated endocytosis of CTLA-4- containing vesicles causes activation of CTLA-4 on the cell surface as a result of stimulatory signals arising from both TCR and CD28:B7 signalling pathway interaction. [24]. Stronger TCR signalling causes increased CTLA-4 to be translocated to the cell surface, and this process works in a graded feedback loop. Full activation of T cells is inhibited by suppression of IL-2 production and cell cycle progression in the case of a net negative signal through CTLA-4:B7 interaction [25]. CTLA-4 is also engaged in immune regulation in various ways. T- cell regulators are essential actors in regulating peripheral tolerance because they regulate the activities of effector T cells [26]. T-cell regulators, unlike effector T cells, express CTLA-4 on a constant basis, which is considered to be crucial for their inhibitory activities [27]. CTLA-4 mutation in Regulatory t cells was shown to affect their inhibitory activities in animal studies [28]. Downregulation of B7 ligands on APCs, which leads to decreased CD28 secondary signal which immune cells depend on to activate an immune response in the presence of an antigen-presenting cell, is one method by which Tregs are considered to regulate effector T cells. CTLA4 has a role in the maintenance of tolerance in autoimmune illnesses including diabetic and thyroid disease, as well as a proclivity for unexpected miscarriage. A single-nucleotide polymorphism in exon 1 of CTLA4 has been linked to vulnerability to autoimmune disorders such as Multiple Sclerosis. Blocking CTLA4 with antibodies inhibits the establishment of resistance, boosts anti-tumor activities, and exacerbates autoimmune disorders.
Due to its distinct interactions with CD28 and CTLA-4, B7.1 and B7.2 play diverse roles in immune regulation. The interaction between B7.1 and CTLA-4 is more affine than the interaction between B7.2 and CTLA-4, but CD28 is anticipated to bind B7.2 more effectively than B7.1. B7.1 homodimers feature CTLA- 4-binding sites that are distant from the B7 dimer interface, allowing for the development of a cross-linked B7-CTLA-4 lattice according to co-crystals of B7.1 with CTLA-4. The findings, on the other hand, support a one- to-one interaction between B7.2 and CD28 [20].
Human melanoma cells are inoculated into an immune-deficient mouse modal to create xenograft models. Malignant cells grow and metastasis via lymphoid tissue and blood arteries after being transplanted subcutaneously into immune-deficient mice, which closely resembles human scenarios [29]. Tumor growth processes, key tumorigenesis routes, pharmacological treatment, bio-availability, and toxicity are all studied using xenograft models. The studies mainly focus on the bioavailability, toxicity, invasive and non-invasive studies of tumor growth mechanism and their pathways. Unfortunately, maintained cancer cell lines are isolated duplicates that are not identical to the parent patient- derived cells. While proliferating in the subcutaneous environment, they may lose some metastasis-promoting signals. This leads to inaccurate clinical outcome estimates and accounts for the majority of clinical trial failures [30].
Xenograft Model
Xenograft model transplantations have used a variety of cell types. C57BL/6J mice, which were triggered by particular chemical reagents, were the most often used cell types. This cell line, also known as the B16 cell line, is known for its proclivity for tumor development, invasion, and metastasis. B16F1 and B16F10 cell lines are two well-known in vivo passaging sub-clones. B16F1 is remarkable for having a limited metastatic potential, thus it may be utilized to examine the progression of primary malignancies [30]. Malignant tumors are implanted into the same species diversity background to create syngeneic models [31,32]. These mice have a completely functioning immune system and are immunological competent. Dendritic cells presenting with tumor-released antigens were shown to facilitate natural interactions between melanocytes and immune cells including T cells and B cells in the investigation of the melanoma microenvironment [33]. B16 models are ideal for an animal in vivo investigations because of their fast development and high turnaround time. Subcutaneous tumors, for example, generally achieve relevant status in two to four weeks [34]. B16F10, on the other hand, has a stronger capacity to spread to distant organs, with the lungs having the highest risk of metastasis [35].
Genetically Modified Models (GMMs)
Through studies of GMMs, we’ve learned a lot about how genes work, which has helped us develop more effective targeted therapies. Melanoma development can be more precisely measured when combined with other neoplasm-inducing methods like UV induction. GMMs are more accurate in predicting medication efficiency than other pre-clinical models [36]. Transgenic mice with altered gene expression specific to melanomagenesis were used to create genetic engineering models [37]. RET he RET gene (ret proto-oncogene), encodes the proto-oncogene tyrosine-protein kinase receptor Ret, a receptor tyrosine kinase (RTK). Melanoma can be accelerated by the expression of the RET gene, Activating point mutations in RET can give rise to hereditary cancer syndrome, The RET proto-oncogene, which codes for glial cell-derived neurotrophic factor-specific receptor tyrosine kinase [38], forms the foundation of this concept. As a result, months later, benign melanoma tumors appear, followed by malignant tumor development and organ spread.
Mouse model strains that have been genetically engineered come with a lot of work and a lot of money. Furthermore, certain genetic changes have negative impacts on reproductive capacity, making genotyping for targeted medication less effective.
Surgical removal and radiation therapy are the first-line treatments for melanoma patients. Wide local excision, lymphadenectomy, and sentinel lymph node (LN) biopsy are all options for surgery. Melanoma surgery can be coupled with chemotherapy, radiation treatment, biologic therapy, and targeted therapy in many situations. [39].
In recent times, immunotherapeutic therapies against melanoma have received much interest. The “checkpoint inhibitors” exhibited from attaching to their partner proteins. This stops the “off” signal from reaching the T cells, allowing them to destroy cancer cells [40]. In 2011, the FDA approved ipilimumab, a monoclonal antibody that targets cytotoxic T-lymphocyte antigen 4 (CTLA-4), followed by the approval of pembrolizumab and Nivolumab, antibodies that target programmed cell death 1 (PD-1) in 2014 [41]. However, additional problems arose quickly as a large number of patients showed either transient or no responses to checkpoint inhibitors, while long-term survival and cure were only obtained in a tiny percentage of patients [42].
Lipoplexes
synthetic lipid transporters of DNA are lipoplexes (cationic liposomes). They may form complexes (spherical and continuous bilayer structures) with negatively charged DNA due to their positive surface charge. Lipoplexes enter cells primarily by clathrin-mediated endocytosis (CME), or fusion with the cell membrane, whereas polyplexes enter cells through caveolae-mediated endocytosis (CvME) [43]. Their job is to shield nucleic acids from enzymatic hydrolysis and to prevent DNA from activating the immune system. Biocompatibility is essential for lipoplexes.
A flip-flap mechanism releases lipoplexes from the endosome. Fusogenic lipids designed to carry DNA or siRNA directly into the cytoplasm in a fusion- dependent way in order to prevent inefficient escape from the endosome and poor transfection effectiveness by bypassing cellular endocytosis (e.g., DOPE) have been employed in certain research to improve transfection efficiency by allowing lipoplexes to merge with both the receptor-mediated endocytosis membrane and release DNA molecules [44]. By transitioning from bilayer to inverted hexagonal structures, DOPE can catalyze the fusion process [45].
In an advanced melanoma model treated with cancer vaccine via encapsulated siRNA, liposomeprotamine- hyaluronic acid (LPH) was discovered to modify a tumor immunosuppressive milieu defined by cytokines like TGF- [46].
Vesicular Systems
Vesicular systems, which are made up of one or more concentric bilayers, are utilized in targeted drug delivery for there localize the drug to the site of action, reducing drug concentrations elsewhere in the body. Moreover, because of their shape, which includes core aqueous compartments surrounding a hydrophobic bilayer structure of phospholipids (liposomes) or surfactants (niosomes), they may transport both hydrophilic and fat-soluble medicines. Furthermore, other techniques, such as size regulation or functional, can be used to extend their drug circulation periods. discovered a chitosan-coated liposomes approach to stabilize and increase Indocyanine green skin penetration, a potential option for topical melanoma photodynamic treatment (PDT). PDT is used to alleviate the inefficiency of NPs induced by the EPR effect (increased permeability and retention). PDT can be used to lower high interstitial pressure and enhance vascular permeability, allowing NPs to be delivered more easily.
For basal cell carcinoma, Rata et al. obtained AS1411 aptamer-functionalized liposomes. The AS1411 aptamer is a 26-mer DNA aptamer that binds to nucleolin, a 76 KDa protein that is extensively produced in melanoma cells’ plasma membrane [41,42,45].
NP’S Based
The importance of implementing polymeric nanoparticles (NP) was to reduce the loss and early degradation of the medicine carried inside them, which normally occurs after chemical enzymatic deactivation. They’ve demonstrated the capacity to improve medication bioavailability, lessen unpleasant side effects, and raise the proportion of drugs stored in a specific part of the body [47, 48]. The introduction of biocompatible nanomedicine delivery devices can help in cancer therapy. Because the majority of cytotoxic medicines discovered for cancer are lipophilic, their anticancer effectiveness is restricted due to adverse pharmacodynamic and pharmacokinetic characteristics. Anticancer drugs have successfully been modified by using the polymers such as PLGA PEG which are hydrophilic has been extensive researches carried out by using the formulation strategies by the accumulation of nanoparticles . polymer NPs, such as nanospheres and nanocapsules, polymer micelles, polymers, dendrimer- based micelles, and polymer-drug conjugates, can be engineered depending on the characteristics of the polymer and their uses [40].
Active targeting
Active targeting is a targeting compound (e.g., ligand, antibody, peptide) that is added to a nanoparticle system, it is used to target particular alterations in cancer cell biology that are substantially elevated in comparison to healthy surrounding cells and tissues. increased delivery system specificity, resulting in reduced unfavorable non-specific interactions and drug localization in peripheral tissues
Nano-Vaccines
In the field of cancer immunotherapy, nano vaccines might be a promising technique. In this regard, Xu et al. developed a method for fabricating a customized nanovaccine based on a cationic fluoropolymer that may be used for cancer immunotherapy after surgery. Connect et al. demonstrated that NPS might be used to treat melanoma in the same way as vaccinations are used to treat viral infections [49]. Melanoma A/MART-1 (A27L) major histocompatibility complex class I (MHCI) restricted peptide (MHCI-ag) and MHCII MHC class I and class II antigen presentation pathways are targeted by Melan-A/MART-1 [47] (MHCII-ag).
Nanogel
Ability to load both small and macromolecules makes it easier to administer numerous therapeutic substances. Nanogels can be given a variety of, based on the polymeric’ dual mode of enabling combined treatment for simultaneous cancer therapy [46]. Entrapping liposomes pharmaceuticals, enhancing the depth of penetration, of drug and attaining a precise target impact appear to be advantages of nanotechnology. PH-sensitive bio-sensing and imaging and the delivery of the drug to the molecular can be obtained by using the different forms of hydrogels. [50]. Nanogel-based protein delivery has been proved to be a viable alternative to standard chemotherapy for cancer vaccine delivery. When poly (I: C) was used as an adjuvant, a significant therapeutic effect was seen [51, 52].
Nanotechnology Drugs in the Current Market
Drugs based on nanotechnology are now available on the market. There are no authorized nanotechnology products for melanoma, including albumin-conjugated and polymeric paclitaxel, liposomal versions of many drugs, Preclinical studies to assess the safety and efficacy of nanoparticulate systems are now underway, and some of these therapies for drug-resistant melanoma may be approved soon.
In conclusion, there are certain challenges to overcome in the development process, Advanced melanoma management remains a major concern, and greater knowledge of cancer biology is required to solve the hurdles that come upon current therapy such as nanoparticulate formulation stability and reproducibility limitations, poor in vitro/in vivo correlation, delay development of suitable animal models, regulatory obstacles, and medication failure in early clinical trials. nanoparticulate systems for drug resistance at the cellular and molecular level. In preclinical investigations, several of these nanoparticles performed well, with excellent pharmacokinetic and pharmacodynamic characteristics. The market success of liposomal formulations shows that the road has been built and, agents. This is mostly owing to the fact that advanced cancer is typically resistant to standard treatments. As a result, potential treatment techniques are needed.
References
- Atezolizumab in advanced hepatocellular carcinoma: good things come to those who wait Rizzo Alessandro, Ricci Angela Dalia, Brandi Giovanni. Immunotherapy.2021;13(8). CrossRef
- A new understanding in the epidemiology of melanoma Erdei Esther, Torres Salina M.. Expert Review of Anticancer Therapy.2010;10(11). CrossRef
- The melanoma skin cancer detection and classification using support vector machine Alquran Hiam, Qasmieh I., Alqudah Ali Mohammad, Alhammouri Sajidah, Alawneh Esra’a, Abughazaleh A., Hasayen Firas. 2017 IEEE Jordan Conference on Applied Electrical Engineering and Computing Technologies (AEECT).2017. CrossRef
- Precision Diagnosis Of Melanoma And Other Skin Lesions From Digital Images. AMIA Jt Summits Transl Sci Proc. 2017;2017:220-226. Published 2017 Jul 26 Bhattacharya A, Young A, Wong A, Stalling S, Wei M, Hadley D. .
- Skin lesion analysis toward accurate detection of melanoma using multistage fully connected residual network Razzak Imran, Shoukat Ghosia, Naz Saeeda, Khan Tariq Mahmood. IJCNN 2020 : Proceedings of the 2020 International Joint Conference on Neural Networks.2020. CrossRef
- “Skin cancer in skin of color.” Dermatology nursing vol. 21,4 (2009): 170-7, 206; quiz 178 Bradford , Porcia T. .
- Regulating water states by vacancies for cancer therapy Wang Han, Gao Hongbo, Jiang Xingwu, Zhao Peiran, Ni Dalong, Tang Zhongmin, Liu Yanyan, Zheng Xiangpeng, Bu Wenbo. Nano Today.2021;37. CrossRef
- Second near-infrared photothermal materials for combinational nanotheranostics Xu Cheng, Pu Kanyi. Chemical Society Reviews.2021;50(2). CrossRef
- Radiotherapy toxicity De Ruysscher Dirk, Niedermann Gabriele, Burnet Neil G., Siva Shankar, Lee Anne W. M., Hegi-Johnson Fiona. Nature Reviews. Disease Primers.2019;5(1). CrossRef
- Immune-related adverse events with immune checkpoint blockade: a comprehensive review Michot J. M., Bigenwald C., Champiat S., Collins M., Carbonnel F., Postel-Vinay S., Berdelou A., Varga A., Bahleda R., Hollebecque A., Massard C., Fuerea A., Ribrag V., Gazzah A., Armand J. P., Amellal N., Angevin E., Noel N., Boutros C., Mateus C., Robert C., Soria J. C., Marabelle A., Lambotte O.. European Journal of Cancer (Oxford, England: 1990).2016;54. CrossRef
- Melanoma: from mutations to medicine Tsao Hensin, Chin Lynda, Garraway Levi A., Fisher David E.. Genes & Development.2012;26(11). CrossRef
- Human skin pigmentation: melanocytes modulate skin color in response to stress Costin Gertrude-E., Hearing Vincent J.. FASEB journal: official publication of the Federation of American Societies for Experimental Biology.2007;21(4). CrossRef
- Discovery of new depigmenting compounds and their efficacy to treat hyperpigmentation: Evidence from in vitro study Lajis Ahmad Firdaus B., Ariff Arbakariya B.. Journal of Cosmetic Dermatology.2019;18(3). CrossRef
- "“Transcription physiology” of pigment formation in melanocytes: central role of MITF." Vachtenheim, Jiri , Jan Borovanský . Experimental dermatology 19.7 (2010).;:617-627.
- "Safety profiles of anti-CTLA-4 and anti-PD-1 antibodies alone and in combination." Boutros , Celine , et al . Nature reviews Clinical oncology 13.8 (2016).;:473-486.
- Inflammatory arthritis and sicca syndrome induced by nivolumab and ipilimumab Cappelli Laura C., Gutierrez Anna Kristina, Baer Alan N., Albayda Jemima, Manno Rebecca L., Haque Uzma, Lipson Evan J., Bleich Karen B., Shah Ami A., Naidoo Jarushka, Brahmer Julie R., Le Dung, Bingham Clifton O.. Annals of the Rheumatic Diseases.2017;76(1). CrossRef
- CTLA-4 and PD-1/PD-L1 blockade: new immunotherapeutic modalities with durable clinical benefit in melanoma patients Ott Patrick A., Hodi F. Stephen, Robert Caroline. Clinical Cancer Research: An Official Journal of the American Association for Cancer Research.2013;19(19). CrossRef
- Evolving Roles for Targeting CTLA-4 in Cancer Immunotherapy Zhao Yinghao, Yang Wei, Huang Yuanyuan, Cui Ranji, Li Xiangyan, Li Bingjin. Cellular Physiology and Biochemistry: International Journal of Experimental Cellular Physiology, Biochemistry, and Pharmacology.2018;47(2). CrossRef
- CTLA-4 and PD-1 Control of T-Cell Motility and Migration: Implications for Tumor Immunotherapy Brunner-Weinzierl Monika C., Rudd Christopher E.. Frontiers in Immunology.2018;9. CrossRef
- Genetic absence of PD-1 promotes accumulation of terminally differentiated exhausted CD8+ T cells Odorizzi Pamela M., Pauken Kristen E., Paley Michael A., Sharpe Arlene, Wherry E. John. Journal of Experimental Medicine.2015;212(7). CrossRef
- Programmed Cell Death 1 (PD-1) and Cytotoxic T Lymphocyte-Associated Antigen 4 (CTLA-4) in Viral Hepatitis Cho Hyosun, Kang Hyojeung, Lee Hwan Hee, Kim Chang Wook. International Journal of Molecular Sciences.2017;18(7). CrossRef
- Anti-CTLA-4 antibody-functionalized dendritic cell-derived exosomes targeting tumor-draining lymph nodes for effective induction of antitumor T-cell responses Phung Cao Dai, Pham Thanh Tung, Nguyen Hanh Thuy, Nguyen Tien Tiep, Ou Wenquan, Jeong Jee-Heon, Choi Han-Gon, Ku Sae Kwang, Yong Chul Soon, Kim Jong Oh. Acta Biomaterialia.2020;115. CrossRef
- "Structures of immune checkpoints: An overview on the CD28-B7 family." Liu, Weifeng , Xingxing Zang . Structural Immunology.2019;:63-87.
- "Co-Signal Molecules in T-Cell Activation." Co-signal Molecules in T Cell Activation (2019): 3-23. Azuma Miyuki. Advances in Experimental Medicine and Biology.2019.
- "Lentiviral delivery of CTLA‑4 shRNA improves the expansion of cytokine‑induced killer cells and enhances cytotoxic activity in vitro." Rui, Tao , et al . Oncology letters 15.1 (2018).;:741-746.
- "CTLA-4 and PD-1 pathways: similarities, differences, and implications of their inhibition." . Buchbinder , Elizabeth I, Anupam Desai . American journal of clinical oncology.2016;39.1:98.
- "Constitutive clathrin-mediated endocytosis of CTLA-4 persists during T cell activation." Qureshi , Omar S, et al . Journal of Biological Chemistry 287.2012;12:9429-9440.
- "Trans-endocytosis of CD80 and CD86: a molecular basis for the cell-extrinsic function of CTLA-4." Qureshi, Omar S, et al . Science 332.;6029(2011):600-603. CrossRef
- Mouse models of metastasis: progress and prospects Gómez-Cuadrado Laura, Tracey Natasha, Ma Ruoyu, Qian Binzhi, Brunton Valerie G.. Disease Models & Mechanisms.2017;10(9). CrossRef
- "Anticancer drug development, a matter of money or a matter of idea." Lu, D. Y., E. H. Chen , T. R. Lu . Metabolomics 5.2.2015;:e134.
- Models of metastasis in drug discovery Talmadge James E.. Methods in Molecular Biology (Clifton, N.J.).2010;602. CrossRef
- Innate and adaptive immune cells in the tumor microenvironment Gajewski Thomas F, Schreiber Hans, Fu Yang-Xin. Nature immunology.2013;14(10). CrossRef
- Ret transgenic mouse model of spontaneous skin melanoma: focus on regulatory T cells Umansky Viktor, Sevko Alexandra. Pigment Cell & Melanoma Research.2013;26(4). CrossRef
- Meeting report: The future of preclinical mouse models in melanoma treatment is now Merlino Glenn, Flaherty Keith, Acquavella Nicolas, Day Chi-Ping, Aplin Andrew, Holmen Sheri, Topalian Suzanne, Van Dyke Terry, Herlyn Meenhard. Pigment Cell & Melanoma Research.2013;26(4). CrossRef
- "Therapeutic molecules for fumigating inflammatory tumor environment." Harikrishnan , Adhikesavan , Vijaykumar Veena . Current Signal Transduction Therapy.2018;13.2:129-152.
- "Generation and characterisation of an estrogen receptor-positive GEMM-derived Pten p53 null transplantable breast tumour model for therapeutic testing." Davies E. J, et al . bioRxiv.2019;:762047.
- Targeted Drug Delivery to Melanoma Liu Qi, Das Manisit, Liu Lydia, Huang Leaf. Advanced Drug Delivery Reviews.2017;127. CrossRef
- CCR5+ Myeloid-Derived Suppressor Cells Are Enriched and Activated in Melanoma Lesions Blattner Carolin, Fleming Viktor, Weber Rebekka, Himmelhan Bianca, Altevogt Peter, Gebhardt Christoffer, Schulze Torsten J., Razon Hila, Hawila Elias, Wildbaum Gizi, Utikal Jochen, Karin Nathan, Umansky Viktor. Cancer Research.2018;78(1). CrossRef
- IL-33/ST2 Axis Regulates Vasculogenic Mimicry via ERK1/2-MMP-2/9 Pathway in Melanoma Yang Fuhan, Wen Mingming, Pan Dayu, Lin Xian, Mo Jing, Dong Xueyi, Liao Shihan, Ma Yuemei. Dermatology (Basel, Switzerland).2019;235(3). CrossRef
- "Soluble NKG2D ligands are biomarkers associated with the clinical outcome to immune checkpoint blockade therapy of metastatic melanoma patients." Maccalli , Cristina , et al . Oncoimmunology 6.;7(2017):e1323618.
- Autoantibodies May Predict Immune-Related Toxicity: Results from a Phase I Study of Intralesional Bacillus Calmette–Guérin followed by Ipilimumab in Patients with Advanced Metastatic Melanoma Da Gama Duarte Jessica, Parakh Sagun, Andrews Miles C., Woods Katherine, Pasam Anupama, Tutuka Candani, Ostrouska Simone, Blackburn Jonathan M., Behren Andreas, Cebon Jonathan. Frontiers in Immunology.2018;9. CrossRef
- "Metastatic melanoma in a 95 years old patient responding to treatment with talimogene laherparepvec followed by nivolumab." Acta Oncologica Naqash, Abdul R, et al . 56.;10(2017):1327-1330. CrossRef
- "Semeval-2015 task 2: Semantic textual similarity, english, spanish and pilot on interpretability." Proceedings of the 9th international workshop on semantic evaluation (SemEval 2015) Agirre, Eneko , et al . 2015. CrossRef
- Kuchroo. "Lag-3, Tim-3, and TIGIT: co-inhibitory receptors with specialized functions in immune regulation." Immunity 44.5 (2016): 989-1004. Anderson, Ana C, Nicole Joller , Vijay K. .
- World-Wide Immunoscore Task Force: meeting report from the “Melanoma Bridge”, Napoli, November 30th–December 3rd, 2016 Galon Jerome, Lugli Alessandro, Bifulco Carlo, Pages Franck, Masucci Giuseppe, Marincola Francesco M., Ascierto Paolo A.. Journal of Translational Medicine.2017;15. CrossRef
- Drug Abuse Treatment through gene manipulation using nanomedicine. Current Pharmacogenomics and Personalized Medicine (Formerly Current Pharmacogenomics) Alavian F. 2018;16(3):182-191.
- Cationic synthetic long peptides-loaded nanogels: An efficient therapeutic vaccine formulation for induction of T-cell responses Kordalivand Neda, Tondini Elena, Lau Chun Yin Jerry, Vermonden Tina, Mastrobattista Enrico, Hennink Wim E., Ossendorp Ferry, Nostrum Cornelus F.. Journal of Controlled Release: Official Journal of the Controlled Release Society.2019;315. CrossRef
- Differential expression of ABCB5 in BRAF inhibitor-resistant melanoma cell lines Xiao J, Egger ME, McMasters KM, Hao H. BMC cancer.2018;18(1):1-10.
- Polysaccharide-based nanogels for drug and gene delivery. In Polysaccharide Carriers for Drug Delivery (pp. 497-557). Woodhead Publishing. 2019 Kumari L, Badwaik HR. .
- Multi-functional core-shell hybrid nanogels for pH-dependent magnetic manipulation, fluorescent pH-sensing, and drug delivery Wu Weitai, Shen Jing, Gai Zheng, Hong Kunlun, Banerjee Probal, Zhou Shuiqin. Biomaterials.2011;32(36). CrossRef
- Graphene Fiber-Based Strain-Insensitive Wearable Temperature Sensor Yu M, Yu G, Dai B. IEEE Sensors Letters.2020;4(10):1-4.
- Convergent Therapeutic Strategies to Overcome the Heterogeneity of Acquired Resistance in BRAFV600E Colorectal Cancer Hazar-Rethinam M, Kleyman M, Han GC, Liu D, Ahronian LG, Shahzade HA, Chen L, Parikh AR, Allen JN, Clark JW, Kwak EL, Faris JE, Murphy JE, Hong TS, Van Seventer EE, Nadres B, Hong CB, Gurski JM, Jessop NA, Dias-Santagata D, Iafrate AJ, Van Allen EM, Corcoran RB. Cancer Discovery.2018;8(4):417-427. CrossRef
License

This work is licensed under a Creative Commons Attribution-NonCommercial 4.0 International License.
Copyright
© Asian Pacific Journal of Cancer Biology , 2021
Author Details