A Preliminary Investigation on the Possibility of Developing a Cancer Vaccine Using Snake Venom Components
Download
Abstract
Cancer is a global health concern and is still the leading cause of death worldwide, with 10 million deaths caused, so far, by the disease in 2020. Although there are treatments to treat some cancers and save lives, these treatments do have drawbacks and can cause side effects. Although various anticancer remedies have been discovered using natural products in the past, none of them went into clinical trials. Scientists still looking for alternative agents, within animal products. Recently venom is gaining attention for its anticancer activities.
Objective: The present study, therefore, aimed to address the theoretical possibility that people bitten by venomous snakes may also develop prophylaxis, i.e., natural immunity, against cancer. And whether snake venom can be used as a vaccine due to their similarities with potential cancer antigens or not.
Method: An immunoinformatic analysis was performed by correlating potential snake venom components’ amino sequence data with different cancer antigens.
Results: Initially, our findings showed that while developed countries have low snakebite rates, they also demonstrated high cancer rates, in contrast to that developing countries, where they have high snakebite rates but low cancer rates. In terms of the immunoinformatic approach, ten cancer antigens have various similarities with five snake venom components. While the amino acid sequence alignment demonstrates similarities below 40%, the antigenicity index scores were found to be high. We predict that an immune system activated in snakebite victims may elicit an immune response against similar epitopes expressed on cancer cells. Hence, victims of snake bites may gain natural immunity against cancer.
Conclusion: This study reports that non-lethal snakebites may play a vital role in protecting individuals from developing cancer in the future. Although it is a pilot study, such a concept and findings will open doors for future work to address this finding.
Introduction
Cancer is a leading cause of death worldwide and, according to the World Health Organisation (WHO), in 2020, there were 10 million deaths caused by cancer, with lung cancer being the most common [1]. In prosperous countries, about 20%, or one in five, will die of cancer. Furthermore, the number of new cases of cancer incidence is continuing to grow worldwide, and this makes cancer a serious problem affecting the health of human society, especially in the Western world [2]. During normal self-renewal, proliferation is an important and vital physiological process for cells to survive, which is required for maintenance of the cellular multi-potency [3]. On the other hand, damaged DNA normally undergoes repair, however, if the process of repair does not take place, the unpaired DNA might lead to carcinogenesis, i.e., the production of cancer cells, and they can be found in all body parts, leading to many cancer types such as lung, colon, breast, and leukaemia [3,4]. Generally, the types of cancer vary between males and females and age groups. For example, the percentage of prostate, lung, and colon cancer is high in men, whereas bronchus and breast cancer are high in women, and leukaemia is commonly associated with children [2].
During the cell cycle, cells undergo apoptosis as a normal physiological process. The regulation of the apoptotic process is very important in maintaining homeostasis, and the loss of the apoptotic ability of the cell is implicated in a wide variety of human diseases. This is initiated by a widevariousnsic or intrinsic signals and is hence critical in several disease processes [3].
Generally, cancers can be benign, especially those stated above, where cancer remains in its original place and does not invade surrounding tissues or spread to other body parts. In parallel, cancers can also be malignant where the tumour can invade the surrounding tissue and spread throughout the body through the lymphatic or circulatory system; known as metastasis [4]. This is due to the activation of oncogenes and/or the deactivation of tumour suppressor genes (TSG) Table 1), leading to uncontrolled cell cycle progression and the inactivation of apoptotic mechanisms [5].
Tumour Suppressor Genes | Associated Cancer | Other related tumours |
p53 | Li-Fraumeni syndrome | >50% of cancers |
Adenomatous polyposis coli | Familial adenomatous polyposis | Colorectal cancer and gastro-intestinal tumours |
BRCA1 and BRCA2 | Familial breast and ovarian cancer | Colorectal and skin cancer |
NF1 | Neurofibromatosis | Gliomas and sarcomas |
ATM | Ataxia telangiectasia | Lymphoreticular malignancies |
RASSF | Many | Many |
CHK2 | Li-Fraumeni syndrome | Carcinomas of the stomach, colon, and endometrium |
Oncogenes and tumour suppressor genes are two classes of genes that are involved in cancer development [6]. Once these genes are activated, they contribute to the growth of cancer cells in ways such as uncontrolled cell division and enhancing the survival of the cancer cell even after treatment [7]. However, oncogenes can possess tens or thousands of mutations, but only a few can lead to cancer, and these can either be point mutations, inversions, amplifications, or deletions, though point mutations are the most common [7].
These mutations are caused by many factors, including hereditary mutations, diet, smoking, and infectious organisms [4]. Despite these mutations, although the body’s immune system can detect and destroy these cancer cells before they progress further, a cancer cell has the ability to evade the immune system. Therefore, this requires treatment to eliminate the cancer cells.
The increasing incidence of cancer has led to a global effort to find many different therapeutic ways, including chemotherapy (Figure 1), radiotherapy (Figure 2), and surgical procedures. The stage of the disease determines the modality of treatment, including surgery, radiation, or chemotherapy.
Figure 1. Mechanism of Chemotherapy Drug Targeting Tumour Cells by Inducing DNA Damage or Inhibiting Cell Division, Leading to the Apoptosis of the Tumour Cell [8].
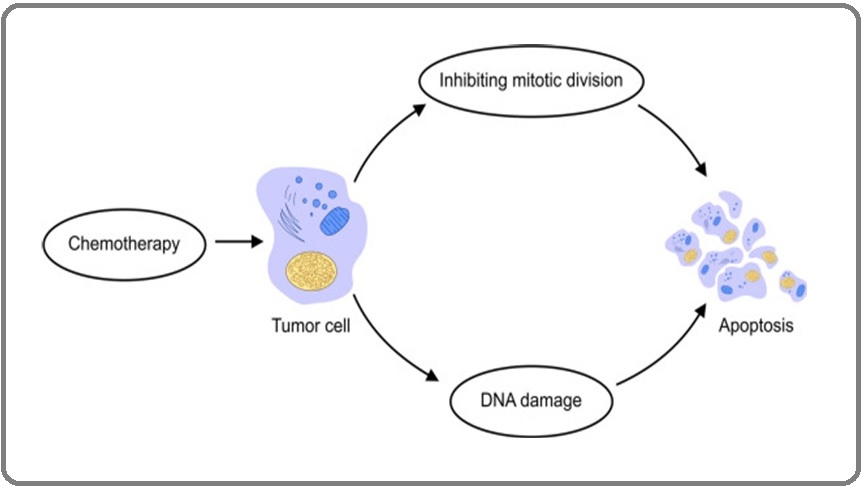
Figure 2. Radiotherapy Treatment Targeting and Destroying Tumour Cells, Releasing Antigens to be Detected by Antigen Presenting Cells and Activate an Immune Response to Destroy the Tumour Cells [9].
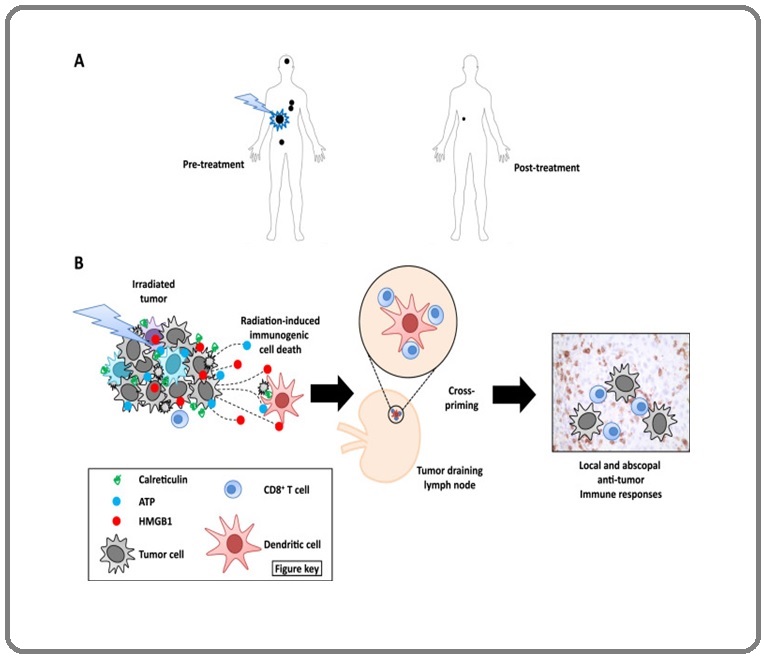
Many of these modalities target signalling pathways responsible for the proliferation of cells and survival. For example, most clinically used cytotoxic anticancer drugs, such as doxorubicin, 5-Fluorouracil, and cisplatin, have the capacity to trigger apoptosis in susceptible cancer cells as well as alternative modes of cell death including Ferroptosis. The combination of all these moderate modalities in treatment increases the therapeutic efficacy but might itself induce a potential risk of toxicity by the drugs [3].
Although surgery, chemotherapy, and radiotherapy are used to treat cancer, many patients are starting to seek other alternatives to treat their cancer, and this includes the use of Complementary and Alternative Medicine (CAM). CAM is used in many countries, such as Europe, the USA, and Asia, and this is because many cancer patients believe that CAM can help cure their disease, reduce the side effects of cancer treatments, and develop immunity to cancer [10]. Furthermore, many patients are using CAM alongside their conventional treatments as they believe it will further increase the chance of curing their disease [11]. CAM involves practices that are not part of conventional medicine, such as massages, diets, prayers, acupuncture, and biological products such as dietary supplements, which include herbal products, vitamins, animal extracts, and prebiotics [12]. Most of the main users of CAM are well-educated, young to middle-aged women. This is due to several reasons which include not trusting conventional treatments as much as their CAM provider; they would rather use natural products that have minimal side effects; or some of their family members and friends have experienced using CAM and it has improved their health and well-being [11]. Despite the fact that CAM seems promising, there is a lack of evidence on how effective CAM is in cancer, whereas conventional medicine has been researched, tested, and gone through clinical trials before being approved to treat patients [13]. More studies are needed to confirm the effects of CAM in treating cancer. Another alternative but strongly evidenced-based treatment for cancer, besides current treatments, is immunotherapy.
Immunotherapy is a burgeoning area that’s generating a lot of interest for its role in treating cancer. Immunotherapy involves harnessing the patient’s innate and adaptive immune response to eliminate cancer cells [14]. This involves enabling the immune system to recognise, target, and eliminate cancer cells in the body, and this involves recognizing certain antigens expressed on cancer cells [15]. Immunotherapy can be carried out by two approaches: passive and active. The passive approach involves administering ex vivo-immune elements such as immune cells or antibodies, which does not initiate an immune response, whereas the active approach involves activating the patient’s immune response, which will then go on to develop specific antibodies and T-cells to eradicate the cancer cells [16]. Currently, there are five cancer immunotherapy treatments: (i) Immune Checkpoint Inhibitors (ICIs), (ii) Adoptive Cell Therapy (ACT) (Figure 3), (iii) Cytokine-based Immunotherapy, (iv) Cancer Vaccines, and (v) Oncolytic Immunotherapy (OI) (Figure 4).
Figure 3. T-cells are Taken from the Patient and Engineered to Attach a CAR. The CAR T-cells are cloned in vitro and are infused back into the patient, where the CAR T-cells will bind to tumour cells and destroy them [17].
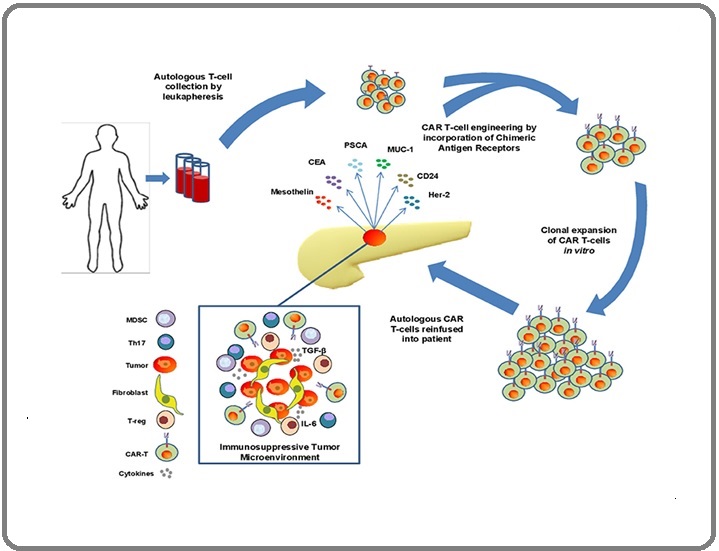
Figure 4. Tumour Cells are Injected with an OV and will Start to Replicate Inside the Tumour Cells. The OV will start to produce antigens on the tumour cells, and this will activate T-cells, initiating an immune response [18].
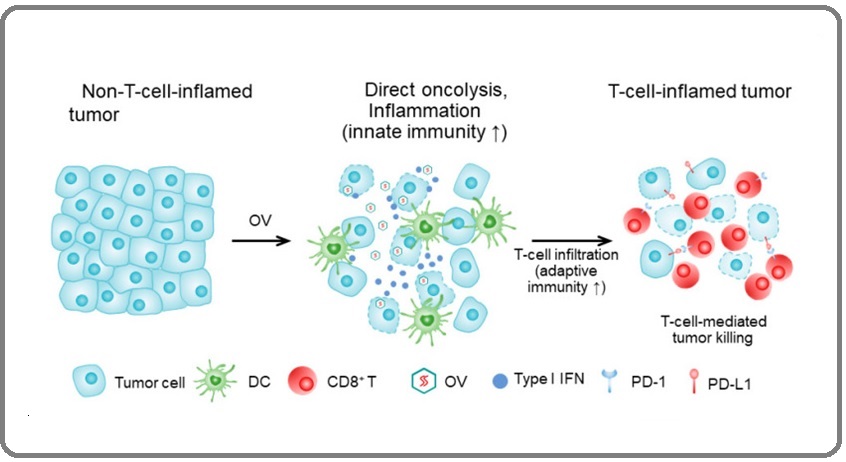
As stated earlier, although new treatments are being developed to treat cancer, many researchers are beginning to look at natural products as an alternative strategy, such as medicinal plant and animal products.
In regard to animals and animal products, researchers are looking into the potential of some animal and insect products such as venom or milk as natural products to treat cancers [3].
An example of an insect product is bee venom. Bee venom is gaining attention for its anticancer activities and is composed of a mixture of peptides such as melittin, adolapin, and apamin; enzymes such as phospholipase A2 and hyaluronidase; and non-peptides such as norepinephrine and dopamine [19]. These components allow bee venom to exert antioxidant, anti- apoptosis, and anti-inflammatory properties, allowing bee venom to be used as a treatment for diseases such as neurodegenerative disease, cardiovascular disease, and rheumatoid arthritis [20]. Furthermore, studies have shown melittin can suppress the ligand-induced phosphorylation of the epidermal growth factor receptor and the human epidermal growth factor receptor 2 in breast cancer cells [21]. However, bee venom has not been approved to treat cancer because melittin is degraded in the body before exerting its anticancer effects. Furthermore, injecting bee venom can cause haemolysis, which is why more investigation is needed to reach clinical trials [19]. Another venom that has been studied to treat cancer is snake venom. Venomous snakes inject their prey with venom through their fangs, and according to the WHO, snakebites are classed as neglected tropical diseases that kill more than 100,000 people each year [22]. Despite this, it is found that snake venom is a potential biological source for the development of therapeutic drugs [22]. Snake venom contains a complex mixture of peptides, proteins, enzymes, carbohydrates, and other bioactive molecules [23]. These proteins express enzymatic activity or non-enzymatic activity. Proteins with enzymatic activity include snake venom metalloproteinase (SVMP), snake venom serine proteinase (SVSP), phospholipase A2 (PLA2), hyaluronidase, L-amino-acid-oxidase, or acetylcholinesterase, whereas non-enzymatic proteins include three-finger toxins, C-type lectins, or these components can cause many toxic metalloproteases such as haemotoxicity, cardiotoxicity, neurotoxicity, or cytotoxicity [23]. Moreover, it is known that snake venom possesses anticancer activity against cancer cells. Studies have shown that PLA2 from Bungarus fasciatus venom can exert cytotoxic effects on human breast cancer MCF7 cells and human lung adenocarcinoma A549 cells [24]. PLA2 is an enzyme with a molecular mass of 13–15 kDa and this enzyme releases lysophospholipids and arachidonic acid through the hydrolysis of the glycerophospholipid membrane [25]. This shows that snake venom has the potential to treat cancer. Another target for snake venom is integrins. Integrins are heterodimeric transmembrane proteins, made by the non-covalent association of α- and β-subunits [26]. Integrins are involved in tumour progression, metastasis, and angiogenesis by upregulating tumour cell invasion, migration, proliferation, and survival [27]. Disintegrins are cysteine-rich, non-enzymatic proteins that are found in the venoms of Elapidae, Viperidae, Crotalidae, and Atractaspididae snake families, and there is evidence that disintegrins can exert antitumour effects involving metastasis dissemination and angiogenesis [28]. Higuchi et al. (2011) [29] cloned a disintegrin, leucurogin, from Bothrops leucurus and found that the disintegrin inhibits the growth of Ehrlich tumours implanted in mice by inhibiting the angiogenesis process of the tumour. Furthermore, Wang et al. (2004) [30] found the disintegrin, admonitor, from Agkistrodon halys brevicaudus teenager, to inhibit platelet aggregation and angiogenesis in ECV304 cells. The effects of snake venom on cancer cells have been well studied for a while now. However, none has ever gone into clinical trials due to its high toxicity in the human body. According to the literature, the components of snake venom may hold the key to curing cancer. This study will investigate if snake venom components could be used in cancer vaccines and if the components could initiate an immune response and generate memory cells that could target developing cancer cells.
Although a sufficient number of studies have been performed on snake venom in vitro, the proclaimed health benefits of such anti-cancer candidates have never been adequately investigated in vivo.
Based on (1) the scientific review literature on the possibility of snake venom as an anti-cancer agent and (2) the preliminary observation that the world’s two distribution maps of both cancer and snake bite were found to be adequately overlapped by showing that while developed countries have low snakebite rates, they also demonstrate high cancer rates, in contrast to that of developing countries, where they have high snakebite rates but low cancer rates (Figure 1 and Figure 2), the present study, therefore, aimed to address this theoretically using bioinformatics tools and elicit the possibility that (i) people who have been bitten by venomous snakes will be developing natural immunity to cancer and hence gain prophylaxis (ii) If that can be proven then components of snake venom could hold a future promise to be used as a vaccine against cancer.
Materials and Methods
Literature search
To support the hypothesis that snake bites may provide prophylaxis and protection for individuals from developing cancer, a background was needed to perform the meta-analysis. A literature search was performed to review any correlation between the number of snakebites and cancer rates in different regions, current studies relating to snakebites, snake venom, cancer, cancer antigens, current cancer therapies and drawbacks, immunotherapies and drawbacks, current cancer immunotherapy and its alternatives. In addition, data on the population in each region were extracted concurrently from the Global Cancer Observatory (GCO) website and the Global Cancer Observatory (iarc.fr). This was used to show the difference in cancer rates between developed and developing countries. In addition, the types of cancer antigens were also collected from the literature as this may show similarities between the snake venom components and the cancer antigens in terms of sequence, structure analysis, etc. This may help in predicting that the generated anti-snake venom antibodies will cross-react with cancer antigens that show antigenic site similarities.
Extracting amino acid sequence data
Following the literature review data, extraction of the amino acid sequence data was initiated to predict if there is a correlation between snake bite and cancer prophylaxis based on sequence and/or structure similarities with the common cancer antigens. To do that, the amino acid sequences of both the snake venom components and the cancer antigens were extracted using the latest NCBI Basic Local Alignment Search Tool (BLAST) search engine. BLAST: Basic Local Alignment Search Tool (nih.gov).
The selection of the amino acid sequences was based on the potent snake venom molecules within the venom of Echis ocellatus including metalloproteinase, serine proteinase, phospholipase A2, hyaluronidase, and C-type lectin. We have selected the Echis ocellatus as one of the most clinically important snakes in Africa, especially in Nigeria and Asia [33]. This may be why the cancer rate in Africa is very low compared to the global cancer incident rate.
In parallel to the above sequence search, we also blast searched the amino acid sequences of the common antigens that are found in cancer cells, including melanoma association antigen A3 (MAGE-A3) and A4, epithelial cell adhesion molecule (EpCAM), carbohydrate antigen 19-9 (CA19-9), carcino-embryonic antigen (CEA), alpha-fetoprotein (AFP), human epidermal growth factor receptor 2 (HER2), Wilm’s tumour protein-1 (WT-1), preferentially expressed antigen of melanoma (PRAME), prostate-specific antigen (PSA), prostatic acid phosphatase (PAP), mucin-1 (MUC-1), cytotoxic T-lymphocyte antigen-4 (CTLA-4), NADPH oxidase 4 (NOX4), translocase of outer mitochondrial membrane 34 (TOMM34), and New York esophageal squamous cell carcinoma-1 (NY-ESO-1). Based on the literature, the above cancer antigens are the common antigens that are found in cancer cells.
Sequence analysis
An alignment of the amino acid sequences of both cancer antigens and the snake venom molecules was performed using the EMBOSS (European Molecular Biology Open Software Suite) Needle alignment tool. EMBOSS Needle < Pairwise Sequence Alignment < EMBL-EBI [34]. The similarity score was used to decide which cancer antigen best matches the snake venom components that may initiate an immune response.
Creating protein structures
Following the sequence analysis, the protein structures of the individual snake venom molecules and the cancer antigen were created using the bioinformatic web-based tool PHYRE2 [PHYRE2 Protein Fold Recognition Server (ic.ac.uk)]. The PHYRE2 is an online tool used to predict and analyse protein structures, mutations, or functions, and this is carried out by submitting the amino acid, or nucleotide, sequence into the software to create a predicted structure of the protein [35]. In our case, the protein structure was created by submitting the extracted amino acid sequence of the cancer antigens obtained from the BLAST search.
Protein 3D structure analysis
To further visualise the protein structure obtained from PHYRE2, the amino acid sequences were further analysed by creating a 3D structure using the UCSF Chimera software [Download UCSF Chimera] that allows visualising and analysis of the molecular structures and their related data. Furthermore, it allows users to interact with their molecular structures to produce a better visual effect [36]. The hydrophobicity surface effect of the created 3D structure was ncluded to give a better image of the cancer antigen proteins. The similarities between the snake venom and cancer antigens were located in the 3D structure using different colours; red indicating single and multiple epitope similarities.
Antigenicity
To further explore whether the similarities between the snake venom molecules and cancer antigens are attributed to the hypothesis of cancer prophylaxis, an antigenic index analysis was performed to predict if the predicted antigenic peptide may reflect the immunogenic similarities between the individual snake venom molecules and the cancer antigens.
The Immunomedicine Group [Immunomedicine Group: Tools] PREDICTED ANTIGENIC PEPTIDES (ucm.es)-an online predicting antigenic peptide tool] This was used to determine the antigenicity of a protein and will help to determine which segments within the amino acid sequence are possible targets to initiate an immune response. The software uses the “Kolaskar and Tongaonkar” antigenicity prediction method, and this method is based on the physiochemical properties of the amino acids and their frequent occurrences in experimental known epitopes [37].
Results
Correlation between snakebite and cancer rates
This study was performed to investigate if there is a correlation between snakebite rates and cancer rates. A literature search was first conducted to evaluate the status of the study hypothesis. In Figure 5, the world map shows Asia and Africa to have the highest number of snakebites compared to other regions such as Australia and South America, whereas the other map illustrated in Figure 6, demonstrates which region has the highest cancer rate in numerical order. The regions that are ranked number one include the USA, Canada, Europe, Asia, and Australia; these countries indicate a high cancer rate compared to Africa and South Asia.
Figure 5. Map Shows the Number of Snakebites WorldWide and the Number of Deaths Caused by Venomous Snakes [31].
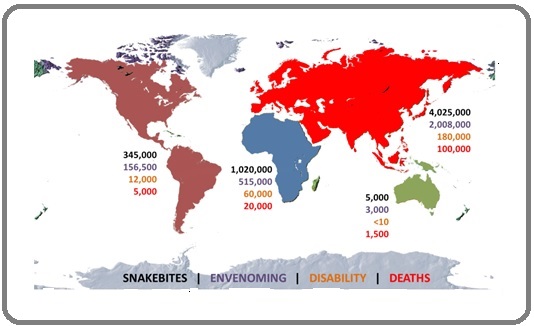
Figure 6. Worldwide Ranking of Deaths Caused by Cancer in 2019. North America, Europe, Asia, and Oceania are number one for the highest deaths caused by cancer. Africa and Southwest Asia are ranked 5th or higher, indicating a low rate of cancer deaths [32].
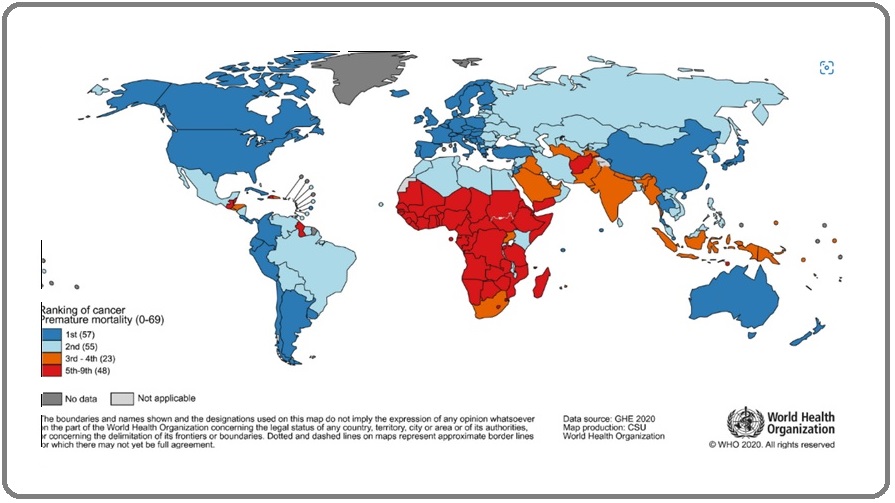
To find the actual figures for the number of cancer cases, the data for the cancer rates in each region across the world were collected from GLOBOCAN and the results are shown in Table 2.
The USA, Europe, Asia, Latin America, and the Caribbean have higher cancer rates than Africa and Australia. The numbers of snakebites and snake envenomations were retrieved from published journals and are shown in Table 3. Asia and Africa have the higher snakebite number per year compared to that found in the USA, Europe, Oceania, Latin America, and the Caribbean. Snake envenomation rates are also higher in Asia and Africa compared to the other regions. The population within each region is also shown in Tables 2 and 3.
Region | Population | Cancer cases per year |
USA | 331,002,653 | 2,281,558 |
Europe | 748,843,410 | 2,339,617 |
Asia | 4,639,847,464 | 9,503,710 |
Africa | 1,340,598,088 | 475,753 |
Oceania | 42,677,809 | 254,291 |
Latin America and Caribbean | 653,962,327 | 1,470,274 |
Region | Population | Number of Snakebites per year | Snake Envenomation per year |
USA | 331,002,653 | 50,000 | 7,000 |
Europe | 748,843,410 | 9,900 | 8,000 |
Asia | 4,639,847,464 | 4,025,000 | 2,000,000 |
Africa | 1,340,598,088 | 1,500,000 | 580,000 |
Oceania | 42,677,809 | 10,000 | 5,000 |
Latin America and Caribbean | 653,962,327 | 345,000 | 150,000 |
It can be seen clearly that both Asia and Africa have the highest populations, reaching over one billion people, in contrast with the rate of cancer and its correlation with the rate of snake bites. This was significant if we correlate the rates of cancer and snake bites against population size in the USA and Europe, taking the population size as a common factor. Collectively, the data for cancer rates, snakebites, and snake envenomation across the globe, specifically within regions of higher rates, were compared with one another and are shown in Figure 7.
Figure 7. Comparison of the Number of Cancers, Snakebites, and Snake Envenomation Per Year. Developed countries illustrate a low rate of snakebites and snake envenomations, whereas developing countries have high incidences of snakebite and snake envenomations. Asia is shown to have a high rate of cancer compared to all the other regions.
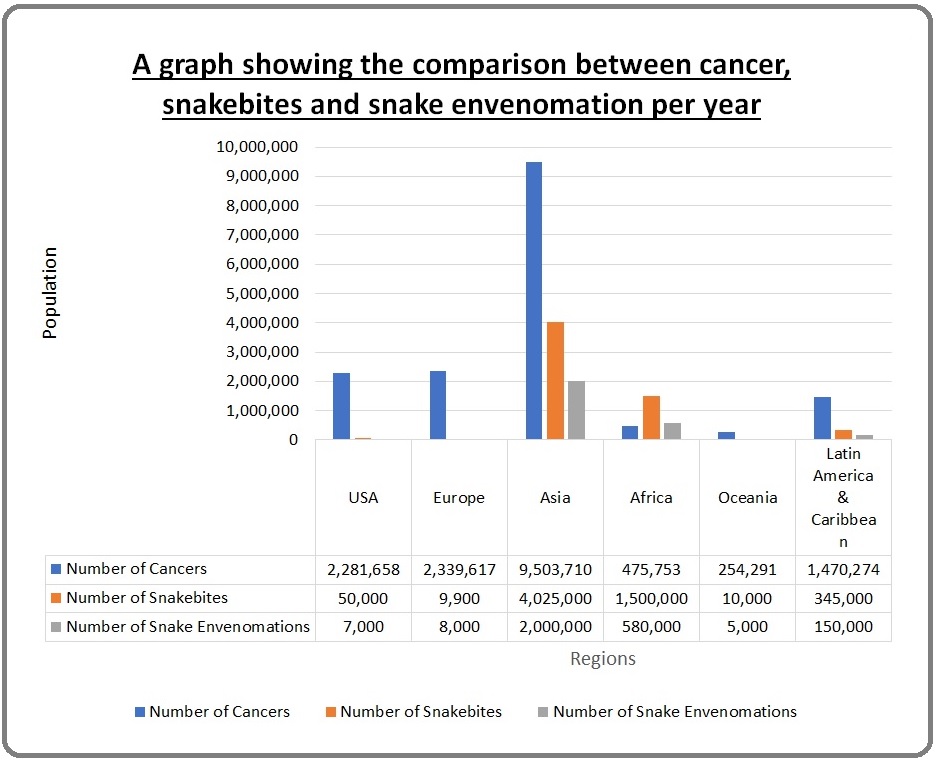
The USA, Europe, Asia, Latin America, and the Caribbean, as well as Oceania, have higher cancer rates per year in parallel with low rates of snakebite and snake envenomation per year.
Similarities between snake venom components and cancer antigens snake venom components
To further explore the correlation between rates of snakebite incidents and cancer, both the snake venom components and cancer antigens were compared for their amino acid and 3D structural alignments and similarities, respectively.
Snake venom Metalloproteinase (SVMP) and Alpha- fetoprotein (AFP) similarities
The SVMP was compared with the AFP and is shown in Figure 8.
Figure 8. Similarities between SVMP and AFP. A) Sequence analysis of metalloproteinase and AFP amino acid sequence being analysed against each other with a similarity score of 25.1%. The red boxes indicate one epitope similarity, and the green boxes indicate more than one epitope similarity. B) Comparison of antigenic profile of both metalloproteinase and AFP. The black box highlights antigenicity relating to the similarities in the sequence analysis. C) 3D structure of the AFP protein highlights the location of the similarities with metalloproteinase. The red areas indicate one epitope similarity, and the green areas indicate multiple epitope similarity.
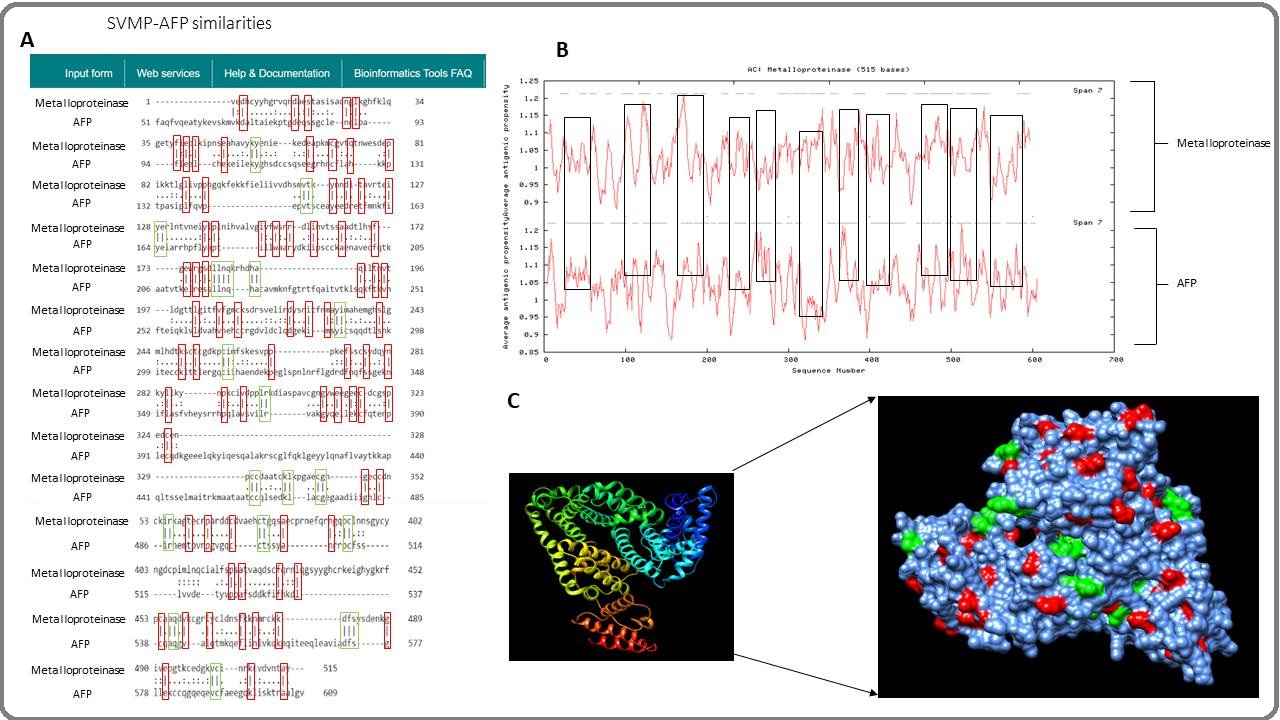
The sequence analysis conducted using the EMBOSS Needle software found a 25.1% similarity between the two proteins, shown in Figure 8A, and most of the similarities are single epitope similarities. The predicted antigenic profile was compared, and both SVMP and AFP show similar antigenicity as most of the amino acid residues are above index 1 (Figure 8B). Most of the antigenicity between both proteins is seen between the sequence numbers 100 to 550. Figure 8C shows the 3D structure, created using the UCSF CHIMERA software, of the AFP protein, highlighting the similar binding locations with the metalloproteinase. The 3D structure shows many binding locations, most of them being single epitopes.
Snake venom Serine Proteinase (SVSP) and Prostate- specific antigen (PSA) similarities
SVSP was compared with PSA for similarities and is shown in Figure 9. The sequence analysis shows a similarity score of 35.1% between the SVSP and PSA (Figure 9A). Most of the similarities are found at the beginning of the amino acid sequence of both proteins. Also, the sequence analysis shows most of the similarities are single epitope similarities. The predicted antigenic profile is shown in Figure 9B, and both proteins show antigenicity between the sequence numbers 100 and 200.
Figure 9C shows the 3D structure of the PSA, highlighting the binding locations that correlate with the sequence analysis.
Figure 9. Similarities between SVSP and PSA. A) Sequence analysis of SVSP and PSA amino acid sequence being analysed against each other, and the similarity score is 35.1%. B) Comparison of antigenic profile of both serine proteinase and PSA and their antigenicity. C) 3D structure of the PSA cancer antigen protein showing the location of the binding site.
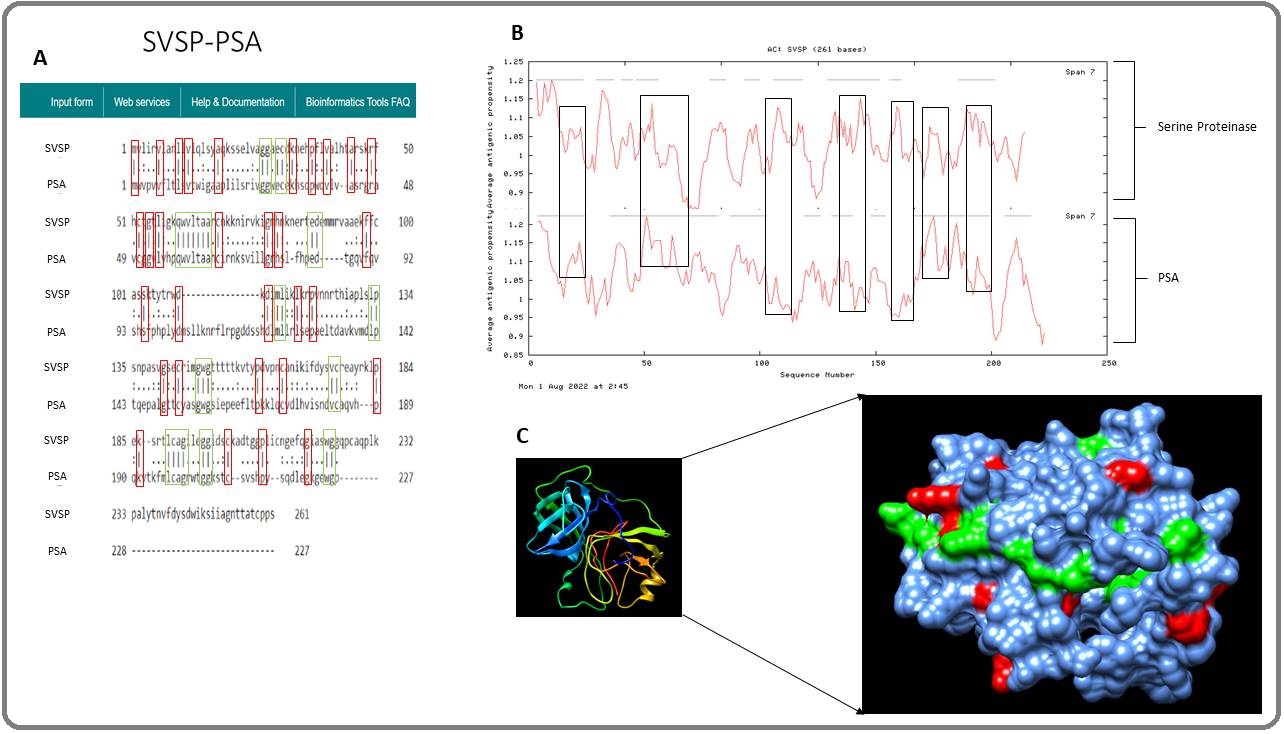
PLA2 and Epithelial cell adhesion molecule (EpCAM) similarities
The snake venom component PLA2 was compared with EpCAM and is shown in Figure 10. The sequence analysis shows a similarity score of 16.42% between the two proteins (Figure 10A).
Figure 10. Similarities between PLA2 and EpCAM. A) Sequence analysis of PLA2 and EpCAM amino acid sequence being analysed against each other, the similarity score is 16.2%. B) Comparison of antigenic profile of both PLA2 and EpCAM. C) 3D structure of the EpCAM protein showing the location of the binding site.
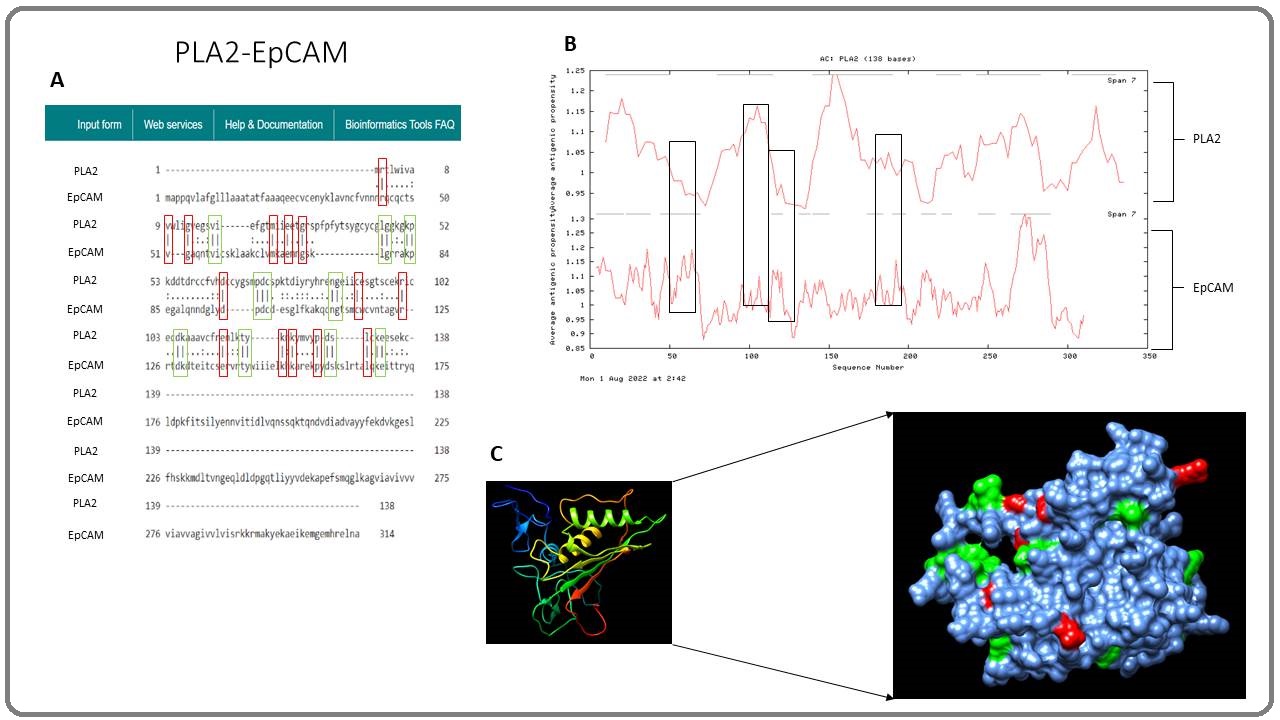
The sequence analysis shows a balance of single and multiple epitope similarity. The predicted antigenic profile was compared and both proteins show antigenicity between the sequence numbers 50 to 200 (Figure 10B). Figure 10C shows the 3D structure of the EpCAM protein, highlighting the binding locations and similarities with PLA2.
Hyaluronidase and AFP similarities
The snake venom component hyaluronidase was compared with the AFP antigen for similarities and is shown in Figure 11. The sequence analysis shows that both the hyaluronidase and AFP amino acid sequences have a similarity score of 18.6% (Figure 11A).
Figure 11. Similarities between Hyaluronidase and AFP. A) Sequence analysis of hyaluronidase and AFP amino acid sequence being analysed against each other, and the similarity score is 18.6%. B) Comparison of the predicted antigenic profile of both Hyaluronidase and AFP. C) 3D structure of the AFP cancer antigen showing the location of the binding site that represents the sequence analysis.
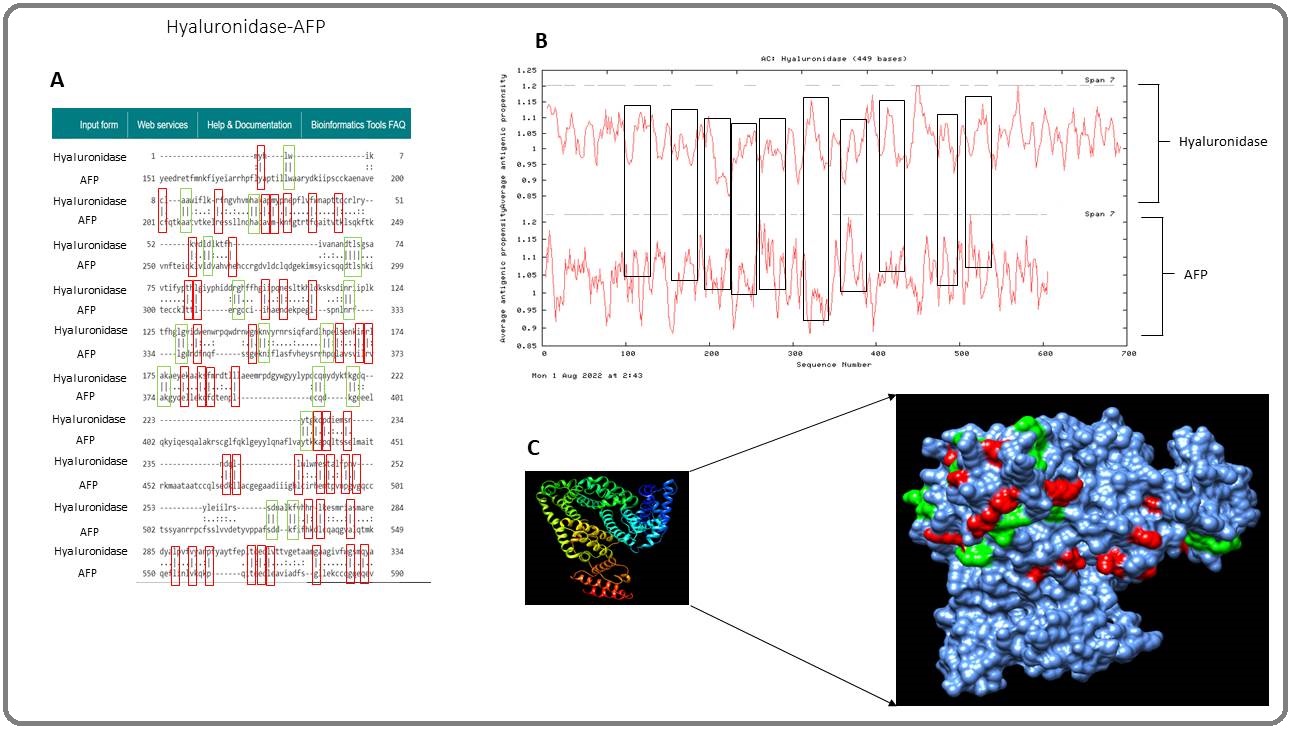
The sequence analysis shows many of the similarities to be single epitopes. The predicted antigenic profile of both proteins is shown in Figure 11B. The antigenic graph shows hyaluronidase to be antigenic like the AFP antigen. The 3D structure of the AFP protein highlights the possible bind locations of antibodies that correlate with the sequence analysis shown in Figure 11C.
C-Type Lectin and PSA similarities
The C-Type lectin was compared with the PSA antigen for similarities and is shown in Figure 12. The sequence analysis shows both C-Type lectin and PSA have a similarity score of 26.0% (Figure 12A).
Figure 12.Similarities between C-Type Lectin and PSA. A) Sequence analysis of C-Type Lectin and PSA amino acid sequence and the similarity score is 26.0%. B) A predicted antigenic profile graph of both proteins showing the antigenicity. C) 3D structure of the PSA cancer antigen showing the location of possible binding sites.
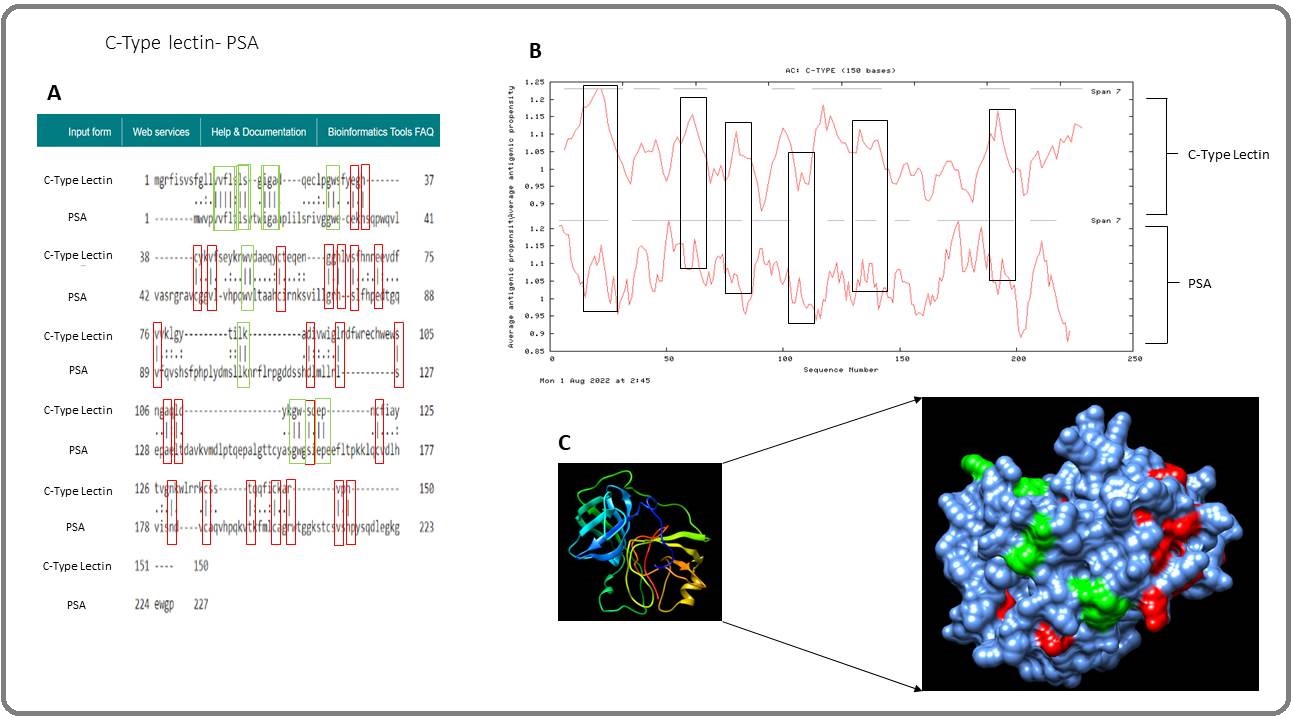
Many similarities are single epitopes. The predicted antigenic profile was created and shows C-Type Lectin to have high antigenicity, as shown in Figure 12B. Both proteins have high antigenicity as both proteins show most of the antigenicity to be above index 1. The 3D structure of the PSA antigen shows the possible binding locations that correlate with the sequence analysis. This is shown in Figure 12C.
Discussion
Cancer continues to remain a global problem, and the rates of people developing cancer are continuing to rise across the globe, leading to more deaths (2). Cancer cells can mutate in any body part and cause different types of cancer, such as lung, breast, liver, stomach, thyroid, and pancreatic cancer (4). Although cancers can be benign or metastatic, there are many treatments that have been used to treat patients with the disease, and these involve current therapies such as surgery, chemotherapy, and radiotherapy. These treatments are used on their own or in combination with one another to maximise their effects on cancer cells [38]. However, these current therapies have many drawbacks, as discussed earlier in Chapter 1.
Although there have been many advancements in the current therapy of cancer, the use of immunotherapy is starting to gain attention among researchers as it involves strengthening the patient’s immune system to destroy cancer cells. However, like current cancer treatments, immunotherapy also has its challenges [14]. Unfortunately, even though there have been many efforts to optimise the current treatments for cancer and increase the survival rates of cancer patients, there are still significant drawbacks that associate with the current treatment that led scientists to look for alternatives, such as natural plants and animal products. In recent years, scientists have been attracted by the potential of animal products, such as camel milk and the nanoparticles within its serum. Furthermore, venom from bees as well as from venomous snakes is under serious research investigation.
One of the most well-studied animal venoms is bee venom. Bee venom has been studied for its antitumor activities and this is due to two components: melittin and PLA2 [19]. There have been many studies showing the effects of melittin on cancer cells. Zhang & Chen (2017) [39] confirm that melittin exerts an anti-angiogenesis effect on lung cancer cells in vitro and in vivo by suppressing the secretion of the vascular endothelial growth factor protein. Furthermore, bee venom is used in China for patients with advanced tumours [40]. In this study, we investigated the possibility that people who have been a victim of a snake bite and survived may gain prophylaxis for developing cancer as observed and predicted in Figure 5 and Figure 6. Based on the map shown in Figure 6, regions such as the USA, Europe, Asia, and Australia are ranked number one for high cancer rates in these areas compared to other regions such as Africa and Southwest Asia. In the US, cancer is the second leading cause of death. This is because the risk of developing cancer increases with age, and over the years the population in the US has increased, especially in the elderly age group. This is confirmed in the literature as many have stated that most cancer incidences are related to age [41]. Moreover, Africa has a low cancer rate and a higher rate of snakebite and snake envenomation compared with other regions. Asia has the highest number of snakebites per year, at over four million, and the highest rate of snake envenomation, at two million per year more than the other regions (Table 3). This has led to the belief that non-lethal snakebites may play a vital role in protecting snakebite victims in Africa and Asia from developing cancer, as the cancer rates are low (Figure 13).
Figure 13. A Venomous Snake Bites their Victim and Inject Parts, or All, of their Venom into their Victim. The snake venom components enter the body and activates the immune system to produce memory B-cells to prevent the development of cancer in the future.
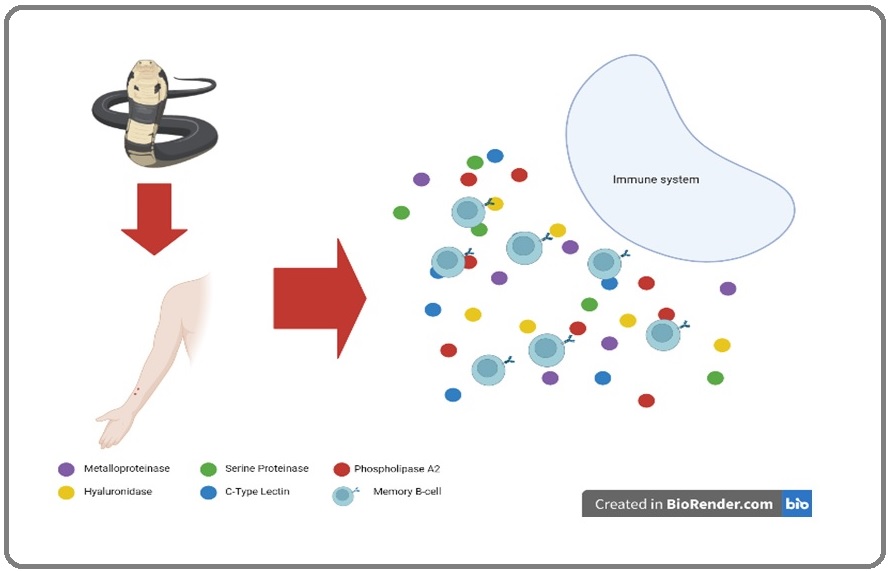
MAGE-A cancer antigens were first identified by T-lymphocytes and many researchers have focused immunotherapies on the antigen and its subfamilies [42]. In adoptive T-cell therapy, the most common antigens that are used are NY-ESO-1 and MAGE family members [43]. In this study, the MAGE-A4 cancer antigen has an amino acid similarity of 18.4% with SVMP (Figure 15). Davari et al. (2021) [44] conducted a study using MAGE-A4 as a target for T-cell-based immunotherapy and found that the T-cell receptor, bbT485, could successfully bind to the MAGE-A4 antigen and kill tumour cells in vitro and in vivo. Ueda et al. (2018) [45] developed a cancer vaccine using the MAGE-A4 antigen for phase I clinical trials and found that 100 µg of the cancer vaccine was able to trigger an immune response in 29% of their patients with advanced or metastatic cancers. This indicates that a small amount of the MAGE-A4 antigen is enough to trigger the immune system. This may explain why non-lethal snakebite victims do not develop cancer, as only small amounts of venom are needed to trigger the immune system and produce memory B-cells,
Another antigen that is used in current immunotherapies is PAP. PAP is an enzyme produced by prostate tissue and is mainly expressed in prostate cancers [46]. In this study, the PAP antigen is shown to have similarities with the SVMP as both the amino acid sequences have a 17.5% similarity (Figure 16). Also, both proteins have similar antigenicity in some parts of the amino acid sequence, indicating that both proteins can initiate an immune response if used as a treatment. PAP is currently being used in immunotherapy to develop a cancer vaccine against prostate cancer. Sipuleucel-T is an autologous cancer vaccine that is approved by the United States Food and Drug Administration to be used on patients with metastatic castration-resistant prostate cancer (mCRPC) and this vaccine targets PAP antigens [47]. Kantoff et al. (2010) [48] confirm that the Sipuleucel-T cancer vaccine can improve the survival rate in men with mCRPC as they found the vaccine to result in a 4.1-month improvement with a 3-year survival rate. This indicates that SVMP could be used as a vaccine against mCRPC and possibly other cancer types. Since the first cancer antigen, MAGE-1, was identified by cytotoxic T-lymphocytes for the destruction of cancer cells, this opened the door for immunotherapies to discover more cancer antigens that can be recognised by T-or B-lymphocytes to release antibodies and attack different cancer cells [49] (Figure 14)(Figure 15)(Figure 16).
Figure 14. Difference in Cancer Development between Non-snakebite Victim Compared with Snakebite/snake Envenomation Victim. A) An individual that has not been bitten by a venomous snake begins to develop cancer, and the cancer cells develop into a tumour. B) snakebite/snake envenomation victim have snake venom in the body. Cancer cells start to develop into a tumour and the snake venom components starts to bind to the cancer cells and initiate the production of memory B-cells, the memory B-cells release antibodies and destroy the cancer cells.
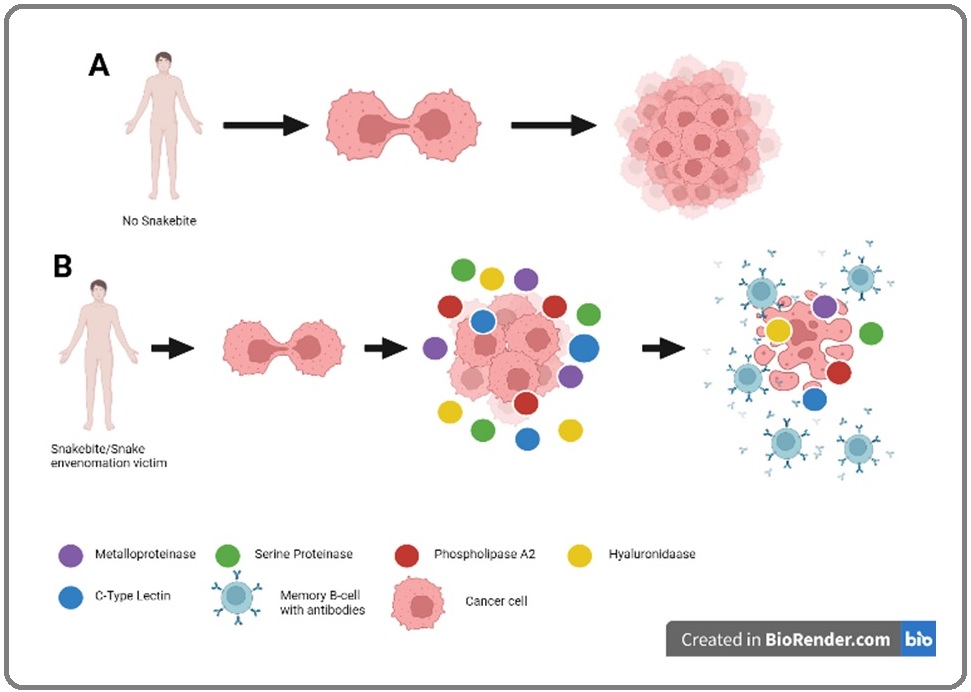
Figure 15. Similarities between SVMP and MAGE-A4. A) Sequence analysis of metalloproteinase and MAGE-A4 amino acid sequence being analysed against each other, and the similarity score is 18.4%. B) Comparison of antigenic profile of both SVMP and MAGE-A4. C) 3D structure of the MAGE-A4 protein shows the location of the similarities with metalloproteinase.
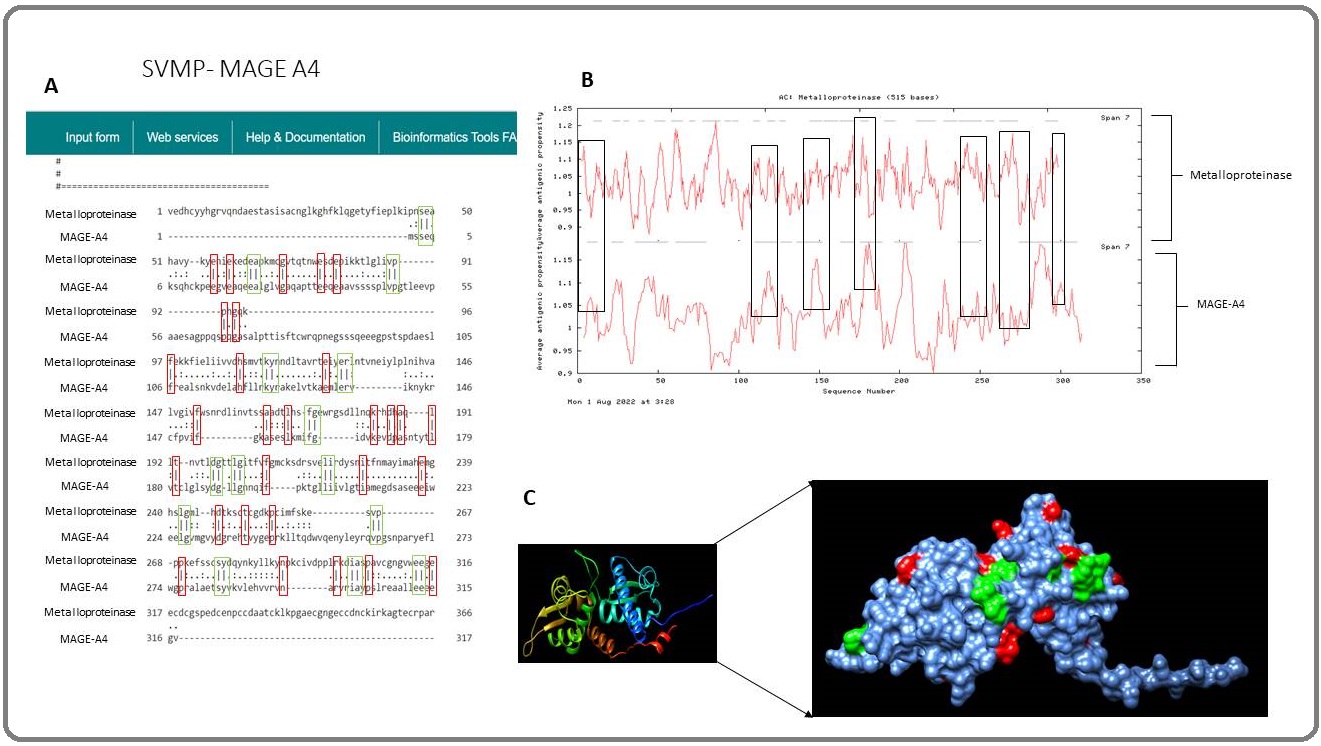
Figure 16. Similarities between SVMP and PAP. A) Sequence analysis of SVMP and PAP amino acid sequence being analysed against each other, and the similarity score is 17.5%. B) Comparison of antigenic profile of both proteins. Boxes indicate antigenicity of both proteins. C) 3D structure of the PAP protein shows the location of the similarities with SVMP, correlating with the sequence analysis. Red areas represent a single epitope and the green areas represent multiple epitopes.
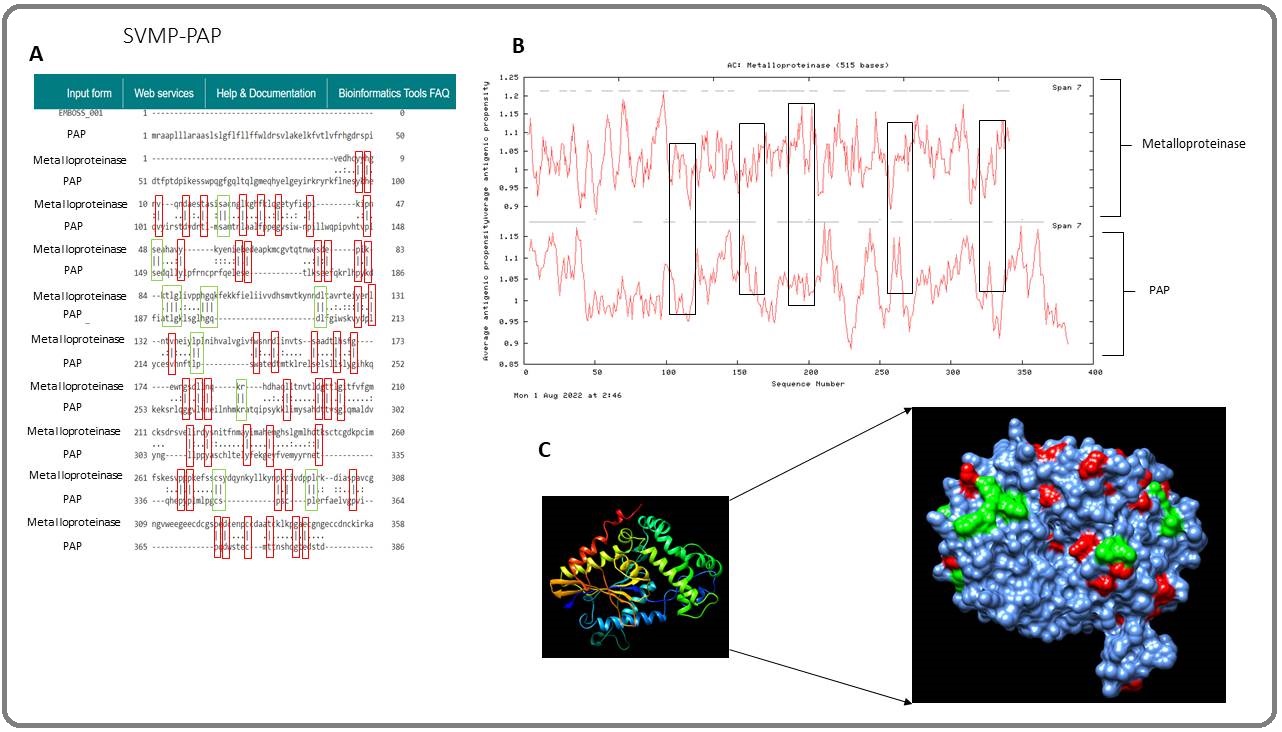
This study has found other cancer antigens that also have similarities with the snake venom components and have not yet been used in therapies but are undergoing clinical trials. AFP is produced by the yolk sac during neonatal life and is a common cancer antigen that is expressed in hepatocellular carcinoma [50]. In this study, the AFP has been shown to have amino acid similarities with two of the snake venom components, SVMP (25.1%) (Figure 10A) and hyaluronidase (18.6%) (Figure 13A). Studies have shown that the AFP antigen could be a potential target for immunotherapy. Cany et al. (2011) [51] carried out AFP-specific immunotherapy that only targets the AFP antigen, and the results showed a 65% reduction in tumour size in mice with hepatocellular carcinoma. Zhu et al. (2018) [52] used the adoptive T-cell approach and genetically engineered T-cells to only recognise the AFP antigens on hepatocellular carcinoma cells, and they found that the AFP antigens were able to redirect the T-cells to recognise and destroy the hepatocellular carcinoma cells. These studies indicate that SVMP and hyaluronidase could be used in immunotherapy and induce a secondary immune response against hepatocellular carcinoma cells. However, there have not been many studies that target the AFP antigen in other cancer types as AFP is also expressed in other cancers such as lung, breast, and gastric cancer [53]. Besides the amino acid sequence of AFP having similarities with SVMP and hyaluronidase, the antigenicity of the proteins appears to be similar (Figure 10B and 13B). Schneider et al. (2016) [54] identified two epitopes in the N-terminal region of the SVMP Atroxlysin-I to be able to induce neutralising antibodies. This shows that SVMP and hyaluronidase may induce the production of memory B-cells due to specific epitopes that are similar to the AFP antigen. This may explain why the cancer rates are low in Africa because snakebite victims may have developed memory B-cells against the SVMP or hyaluronidase molecule, though further investigation is needed to confirm this.
PSA is another cancer antigen that is expressed in prostate cancer and is FDA-approved as a diagnostic biomarker for prostate cancer. Studies have shown PSA to be used in immunotherapy to induce an immune response against prostate cancer. Kubler et al. (2015) [55] conducted a phase I/IIa study to evaluate the immunogenicity and safety of a CV9103 vaccine, which uses mRNA that encodes the PSA antigen, and found the CV9103 vaccine to be immunogenic in patients with castration-resistant prostate cancer. Furthermore, the authors also confirmed that the CV9103 vaccine showed a good safety profile in cancer patients. In the present study, PSA is shown to have amino acid similarities with four of the snake venom components: SVSP (Figure 11), hyaluronidase (Figure 17), PLA2 (Figure 18), and C-Type Lectin (Figure 14), and each snake venom component shows high immunogenicity, which correlates with the PSA antigen.
Figure 17. Similarities between PLA2 and PSA. A) Sequence analysis of PLA2 and PSA amino acid sequence being analysed against each other, and the similarity score is 9.9%. B) Comparison of antigenic profile of both proteins. C) 3D structure of the PSA cancer antigen showing the location of the binding site.
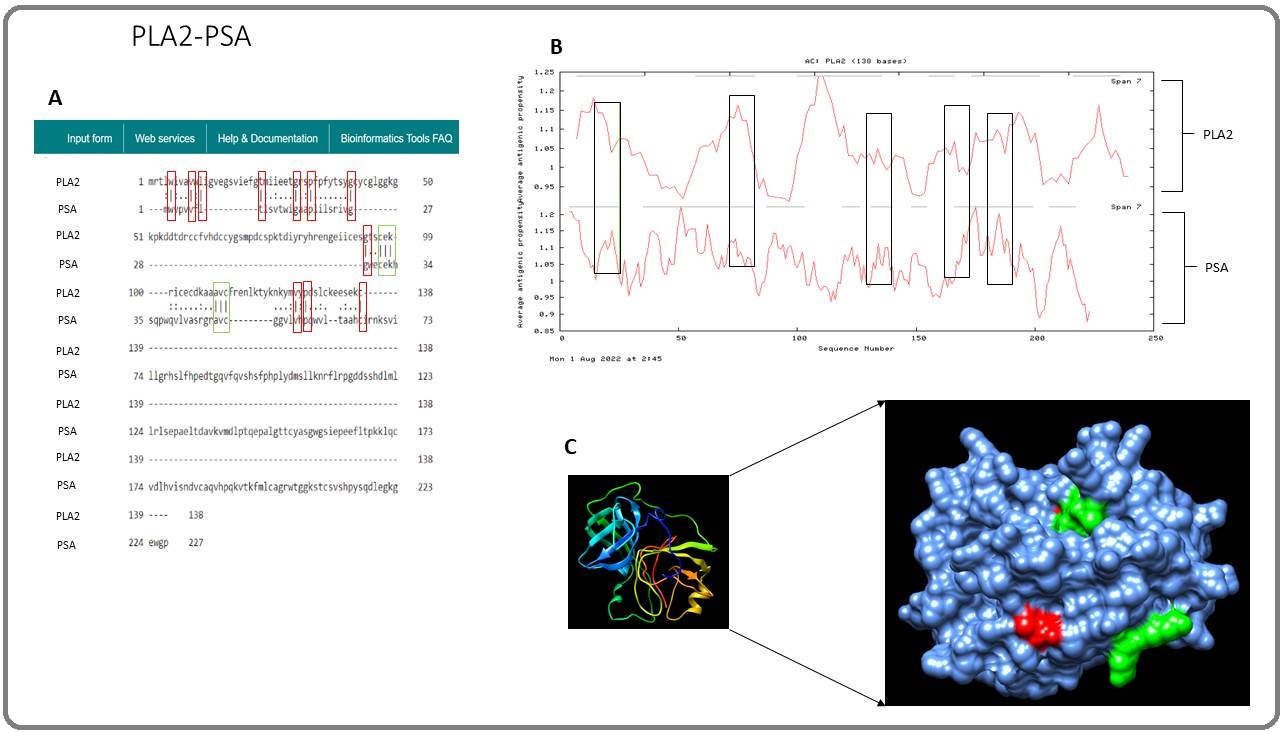
Figure 18. Similarities between Hyaluronidase and PSA. A) Sequence analysis of hyaluronidase and PSA amino acid sequence, and the similarity score is 16.1%. B) A predicted antigenic profile graph of both proteins showing the antigenicity. C) 3D structure of the PSA cancer antigen showing the location of the binding site that correlates with the sequence analysis.
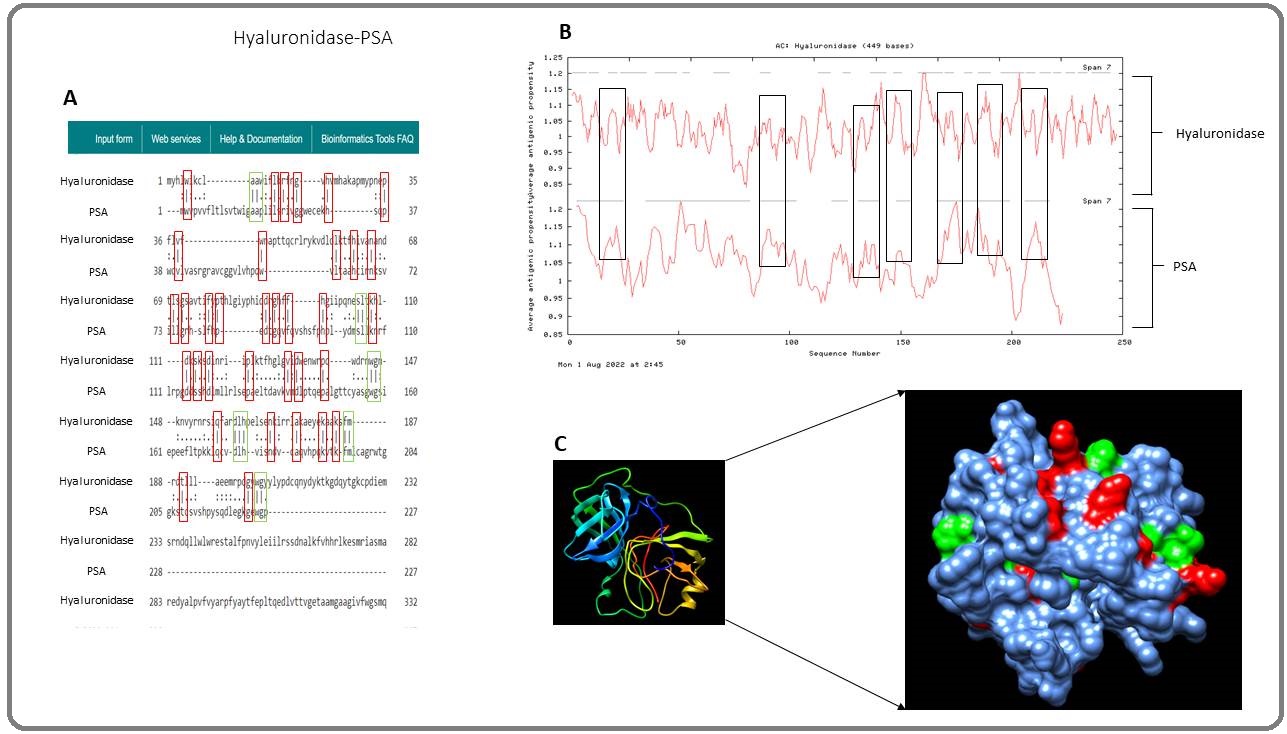
This was further analyzed in the 3D structure. These results point towards the possibility that the four snake venom components may activate the adaptive immune response to produce memory B-cells that target prostate cancer as the number of people with prostate cancer in Africa is 93,173 (World Health Organization, Africa, 2020) [56] compared to the USA where the number of people with prostate cancer is 209,512 (World Health Organization, United States of America, 2020) [57].
Another cancer antigen that is shown to have similarities with snake venom components is the glycoprotein EpCAM. EpCAM has been used as a biomarker for breast cancer as it is overexpressed in breast cancer cells, although it is also expressed in adenocarcinomas of the prostate, pancreas, colon, and stomach [58]. Studies show EpCAM could be used in immunotherapies. Ang et al. (2017) [59] developed an anti- EpCAM T-cell using the CAR-T-cell method and found the modified CAR-T-cells were able to target EpCAM- positive tumours and exert antitumour activity on human ovarian cancer cell lines and mouse models with human ovarian cancer and human rectal tumours. Moreover, the study also found that when injecting the mice repeatedly with the T-cells expressing the anti-EpCAM CAR, the progression of the disease was delayed, meaning that the growth of the tumour can be stopped. In our study, the EpCAM antigen is shown to have amino acid similarities with the snake venom components PLA2 (Figure 12A) and C-Type Lectin (Figure 19).
Figure 19. Similarities between C-Type Lectin and EpCAM A) Sequence analysis of C-Type Lectin and EpCAM amino acid sequence and the similarity score is 15.2%. B) A predicted antigenic profile graph of both proteins showing their antigenicity. C) 3D structure of the EpCAM cancer antigen showing the possible binding locations.
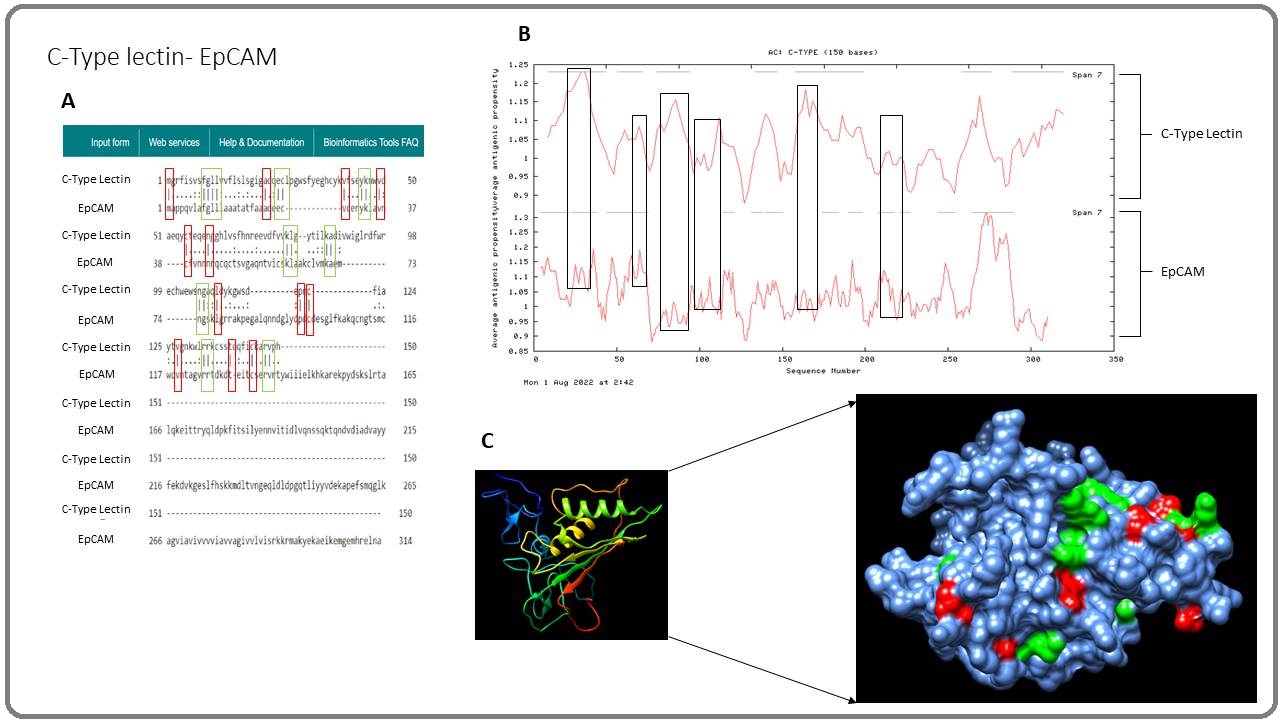
This could be why developing countries have low cancer rates as individuals may have developed memory B-cells from multiple snakebites over a period of time, preventing the development of cancer. Moreover, high levels of PLA2 are commonly found in Elapidae, Viperidae, and Hydrophiidae families, and these snakes are commonly found in Africa and Asia. However, further studies are needed to prove this theory as there are not many published papers regarding snakebites or snake envenomation.
Although this study indicates that non-lethal snakebites may provide protection against developing cancer, there are some considerations. One of the considerations is regarding the cancer rate in Asia (Table 2) as the cancer rate in Asia is high compared to other regions such as the USA, Europe, and Oceania. This was unexpected as these figures did not correlate with the two maps shown in Figure 5 and 6. However, this could be because Asia has a higher population, with over four billion people, compared to the USA and Europe, where there are less than one billion people. So, as a percentage, the cancer rate in Asia is 0.20%, the USA is 0.69%, and Europe is 0.31%. This indicates that Asia has a low cancer rate compared to the USA and Europe based on population size.
Despite the cancer rates may not correlate with the two maps, it should be noted that the number of snakebites (Table 3) does not reflect the true number of snakebites in humans. The reason for this is that most of the true figures of snakebite-related victims cannot reach medical centres or hospitals due to living in remote areas that are miles away. This includes poor farmers and vulnerable communities [60]. Moreover, the cost of traveling is also a factor that prevents snakebite victims from going to the hospital or medical centres as they cannot afford to travel [60]. Another factor that could affect the actual snakebite figures is that snakebite victims are not well-educated in what to do when they are bitten, and this causes them to go to their local healers rather than medical doctors. Therefore, the number of snakebites does not reflect the true figures behind what are shown in Table 3. While the figures reflected in Europe, the USA and Oceania are the actual figures. This is one of the limitations this study faces, which is why future epidemiology studies must be performed in such countries to gather actual figures. If this is carried out, then this can enhance our hypothesis and determine whether non-lethal snakebite is the cause of the low cancer rate in developing countries.
Although it is well known that high sequence similarities can give an indication of some antigenicity, in some cases, this is not always the case. This is true when we generate antibodies against viper snakes such as the Echis ocellatus as they have high amino acid similarities [33], no cross neutralisation was achieved with some other viper species. Therefore, even though the similarities between the individual snake venom components and individual cancer antigens do not possess high sequence similarities, we can still make an argument that the small proportion of the similarities between the cancer antigens and snake venom components may be related to the potent antigenic index that may induce an immune response. Hence, the snake venom components can generate memory B-cells specific to these similarities that can inhibit the correlated function of the molecule. For example, the antigenic similarity may refer to the functional prospect of the molecule or correlate to the binding site of the molecules. The blocking of these binding sites can play a vital role in inhibiting either their function and/or binding mechanism. However, snake venom components must overcome the innate immune response before activating adaptive immunity for a vaccine to be effective [61].
In conclusion, this work is the first epidemiological study to determine whether non-lethal snakebites play a role in protecting individuals from developing cancer. Based on these results, snake venom components showed similarities with potential cancer antigens, and this may play a role in activating the immune system and producing memory B-cells in snakebite victims, thus, protecting them from developing cancer in the future. This indicates that snake venom could possibly be used as a cancer vaccine but, further in vitro and in vivo research is needed to confirm this theory. Although a pilot study, these predictive findings suggest the need for any type of future work to be carried out in this area to prove the hypothesis.
References
- WHO. (2022). Cancer. World Health Organisation. https://www.who.int/news-room/fact-sheets/detail/cancer..
- Review of cancer from perspective of molecular Hassanpour SH , Dehghani M. Journal of Cancer Research and Practice.2017;4(4). CrossRef
- In Vitro Apoptosis Triggering in the BT-474 Human Breast Cancer Cell Line by Lyophilised Camel's Milk Hasson SSAA , Al-Busaidi JZ , Al-Qarni ZAM , Rajapakse S., Al-Bahlani S, Idris MA , Sallam TA . Asian Pacific journal of cancer prevention: APJCP.2015;16(15). CrossRef
- The Development and Causes of Cancer Cooper GM . The Cell: A Molecular Approach. 2nd edition.2000.
- Cancer development, progression, and therapy: an epigenetic overview Sarkar S, Horn G, Moulton K, Oza A, Byler S, Kokolus S, Longacre M. International Journal of Molecular Sciences.2013;14(10). CrossRef
- The biology of cancer Nenclares P., Harrington K. J.. Medicine.2020;48(2). CrossRef
- Oncogenes and tumor suppressor genes in breast cancer: potential diagnostic and therapeutic applications Osborne C, Wilson P, Tripathy D. The Oncologist.2004;9(4). CrossRef
- Immunotherapy. Bio-Engineering Approaches to Cancer Diagnosis and Treatment Shahidian A, Ghassemi M, Mohammadi J, Hashemi M. 2020. CrossRef
- Radiotherapy and Immunogenic Cell Death. Seminars in Radiation Oncology Golden E, Apetoh L. 2015. CrossRef
- A systematic review of complementary and alternative medicine in oncology: Psychological and physical effects of manipulative and body-based practices Calcagni N, Gana K, Quintard B. PloS One.2019;14(10). CrossRef
- Prevalence and reasons for intentional use of complementary and alternative medicine as an adjunct to future visits to a medical doctor for chronic disease Kristoffersen AE , Stub T, Musial F, Fønnebø V, Lillenes O, Norheim AJ . BMC complementary and alternative medicine.2018;18(1). CrossRef
- Complementary and alternative medicine use in patients before and after a cancer diagnosis Buckner C. A., Lafrenie R. M., Dénommée J. A., Caswell J. M., Want D. A.. Current Oncology (Toronto, Ont.).2018;25(4). CrossRef
- Complementary and Alternative Healthcare: Is it Evidence-based? Tabish SA . International Journal of Health Sciences.2008;2(1).
- Recent Advances and Next Breakthrough in Immunotherapy for Cancer Treatment Yang L, Ning Q, Tang S. Journal of Immunology Research.2022;2022. CrossRef
- Neoantigen: A New Breakthrough in Tumor Immunotherapy Zhang Z, Lu M, Qin Y, Gao W, Tao L, Su W, Zhong J. Frontiers in Immunology.2021;12. CrossRef
- Principles of Immunotherapy: Implications for Treatment Strategies in Cancer and Infectious Diseases Naran K, Nundalall T, Chetty S, Barth S. Frontiers in Microbiology.2018;9. CrossRef
- The Potential of CAR T Cell Therapy in Pancreatic Cancer Akce M, Zaidi MY , Waller EK , El-Rayes BF , Lesinski GB . Frontiers in Immunology.2018;9. CrossRef
- Oncolytic Virus Immunotherapy: Showcasing Impressive Progress in Special Issue II Guo Z. Biomedicines.2021;9(6). CrossRef
- Cancer therapeutics inspired by defense mechanisms in the animal kingdom Noble K, Rohaj A, Abegglen L, Schiffman J. Evolutionary Applications.2020;13(7):1681-1700. CrossRef
- Current status and future directions of cancer immunotherapy Zhang H, Chen J. Journal of Cancer.2018;9(10). CrossRef
- Honeybee venom and melittin suppress growth factor receptor activation in HER2-enriched and triple-negative breast cancer Duffy C, Sorolla A, Wang E, Golden E, Woodward E, Davern K, Ho D, Johnstone E, Pfleger K, Redfern A, Iyer KS , Baer B, Blancafort P. NPJ precision oncology.2020;4. CrossRef
- Snake Venoms in Cancer Therapy: Past, Present and Future Li L, Huang J, Lin Y. Toxins.2018;10(9). CrossRef
- Applications of snake venoms in treatment of cancer Shanbhag VKL . Asian Pacific Journal of Tropical Biomedicine.2015;5(4). CrossRef
- Phospholipase A2 from krait Bungarus fasciatus venom induces human cancer cell death in vitro Tran TV , Siniavin AE , Hoang AN , Le MTT , Pham CD , Phung TV , Nguyen KC , Ziganshin RH , Tsetlin VI , Weng C, Utkin YN . PeerJ.2019;7. CrossRef
- Snake Venom Components: Tools and Cures to Target Cardiovascular Diseases Frangieh J, Rima M, Fajloun Z, Henrion D, Sabatier J, Legros C, Mattei C. Molecules.2021;26(8). CrossRef
- Disintegrins from snake venoms and their applications in cancer research and therapy Arruda Macêdo JK , Fox JW , Souza Castro M. Current Protein & Peptide Science.2015;16(6). CrossRef
- Integrins in cancer: biological implications and therapeutic opportunities Desgrosellier JS , Cheresh DA . Nature Reviews. Cancer.2010;10(1). CrossRef
- Effects of Animal Venoms and Toxins on Hallmarks of Cancer Chaisakul J, Hodgson WC , Kuruppu S, Prasongsook N. Journal of Cancer.2016;7(11). CrossRef
- Leucurogin, a new recombinant disintegrin cloned from Bothrops leucurus (white-tailed-jararaca) with potent activity upon platelet aggregation and tumor growth Higuchi D. A., Almeida M. C., Barros C. C., Sanchez E. F., Pesquero P. R., Lang E. a. S., Samaan M., Araujo R. C., Pesquero J. B., Pesquero J. L.. Toxicon: Official Journal of the International Society on Toxinology.2011;58(1). CrossRef
- Cloning and Characterization of Adinbitor, a Novel Disintegrin from the Snake Venom of Agkistrodon halys brevicaudus stejneger Wang J.-H , Wu Y, Ren F, Lu L, Zhao B.-C . Acta Biochimica et Biophysica Sinica.2004;:425-429. CrossRef
- CDN. (2017). The Case for Snakebite Envenoming. Recommendations for the Adoption of an Additional Disease as a Neglected Tropical Disease doi:https://cdn.who.int/media/docs/default-source/ntds/snakebite-envenoming/recommendation-for-snakebite-envenoming-for-adoption-of-additional-ntd.pdf?sfvrsn=c5c37234_4..
- Global Cancer Statistics 2020: GLOBOCAN Estimates of Incidence and Mortality Worldwide for 36 Cancers in 185 Countries Sung H, Ferlay J, Siegel RL , Laversanne M, Soerjomataram I, Jemal A, Bray F. CA: a cancer journal for clinicians.2021;71(3). CrossRef
- Serine protease variants encoded by Echis ocellatus venom gland cDNA: Cloning and sequencing analysis Hasson S. S., Mothana R. A., Sallam T. A., Al-Balushi M. S., Rahman M. T., Al-Jabri A. A.. BioMed Research International.2010;2010(134232). CrossRef
- EMBL-EBI. (2022). EMBOSS Needle Help and Documentation. EMBOSS. doi:https://www.ebi.ac.uk/seqdb/confluence/display/JDSAT/EMBOSS+Needle+Help+and+Documentation..
- The Phyre2 web portal for protein modeling, prediction and analysis Kelley LA , Mezulis S, Yates CM , Wass MN , Sternberg MJE . Nature Protocols.2015;10(6). CrossRef
- Enhancing UCSF Chimera through web services Huang CC , Meng EC , Morris JH , Pettersen EF , Ferrin TE . Nucleic Acids Research.2014;42(Web Server issue). CrossRef
- A semi-empirical method for prediction of antigenic determinants on protein antigens Kolaskar A. S., Tongaonkar P. C.. FEBS letters.1990;276(1-2). CrossRef
- New approaches and procedures for cancer treatment: Current perspectives Debela DT , Muzazu SG , Heraro KD , Ndalama MT , Mesele BW , Haile DC , Kitui SK , Manyazewal T. SAGE Open Medicine.2021;9. CrossRef
- Melittin exerts an antitumor effect on non‑small cell lung cancer cells Zhang S, Chen Z. Molecular Medicine Reports.2017;16(3). CrossRef
- Anti-PD-1 Immunotherapy and Bee Venom for Relapsed and Refractory Liposarcoma: A Case Report Yang W, Zhang Y, Yang G, Geng Y, Chen D, Wang J, Ye Y, Wang H, Xia D, Hu F, Jiang J, Xu X. Frontiers in Oncology.2021;11. CrossRef
- Age and cancer risk: a potentially modifiable relationship White MC , Holman DM , Boehm JE , Peipins LA , Grossman M, Henley SJ . American Journal of Preventive Medicine.2014;46(3 Suppl 1). CrossRef
- MAGE-A Antigens and Cancer Immunotherapy Zajac P, Schultz-Thater E, Tornillo L, Sadowski C, Trella E, Mengus C, Iezzi G, Spagnoli GC . Frontiers in Medicine.2017;4. CrossRef
- Targeting neoantigens for cancer immunotherapy Zhao X, Pan X, Wang Y, Zhang Y. Biomarker Research.2021;9(1). CrossRef
- Development of a CD8 co-receptor independent T-cell receptor specific for tumor-associated antigen MAGE-A4 for next generation T-cell-based immunotherapy Davari K, Holland T, Prassmayer L, Longinotti G, Ganley KP , Pechilis LJ , Diaconu I, Nambiar PR , Magee MS , Schendel DJ , Sommermeyer D, Ellinger C. Journal for Immunotherapy of Cancer.2021;9(3). CrossRef
- NY-ESO-1 antigen expression and immune response are associated with poor prognosis in MAGE-A4-vaccinated patients with esophageal or head/neck squamous cell carcinoma Ueda S, Miyahara Y, Nagata Y, Sato E, Shiraishi T, Harada N, Ikeda H, Shiku H, Kageyama S. Oncotarget.2018;9(89). CrossRef
- Sipuleucel-T for the treatment of prostate cancer: novel insights and future directions Handy CE , Antonarakis ES . Future Oncology (London, England).2018;14(10). CrossRef
- Therapeutic metastatic prostate cancer vaccines: lessons learnt from urologic oncology Lasek W, Zapała Ł. Central European Journal of Urology.2021;74(3). CrossRef
- Sipuleucel-T immunotherapy for castration-resistant prostate cancer Kantoff PW , Higano CS , Shore ND , Berger ER , Small EJ , Penson DF , Redfern CH , Ferrari AC , Dreicer R, Sims RB , Xu Y, Frohlich MW , Schellhammer PF . The New England Journal of Medicine.2010;363(5). CrossRef
- Immune responses to tumour antigens: implications for antigen specific immunotherapy of cancer Jager D, Jager E, Knuth A. Journal of Clinical Pathology.2001;54(9):669-674. CrossRef
- A novel α-fetoprotein-derived helper T-lymphocyte epitope with strong immunogenicity in patients with hepatocellular carcinoma Tamai T, Mizukoshi E, Kumagai M, Terashima T, Iida N, Kitahara M, Shimakami T, Kitamura K, Arai K, Yamashita T, Sakai Y, Yamashita T, Honda M, Fushimi K, Kaneko S. Scientific Reports.2020;10. CrossRef
- AFP-specific immunotherapy impairs growth of autochthonous hepatocellular carcinoma in mice Cany J, Barteau B, Tran L, Gauttier V, Archambeaud I, Couty J, Turlin B, Pitard B, Vassaux G, Ferry N, Conchon S. Journal of Hepatology.2011;54(1). CrossRef
- Identification of α-fetoprotein-specific T-cell receptors for hepatocellular carcinoma immunotherapy Zhu W, Peng Y, Wang L, Hong Y, Jiang X, Li Q, Liu H, Huang L, Wu J, Celis E, Merchen T, Kruse E, He Y. Hepatology (Baltimore, Md.).2018;68(2). CrossRef
- Regulating tumor suppressor genes: post-translational modifications Chen L, Liu S, Tao Y. Signal Transduction and Targeted Therapy.2020;5(1). CrossRef
- Identification of protective B-cell epitopes of Atroxlysin-I: A metalloproteinase from Bothrops atrox snake venom Schneider F. S., Almeida Lima S., Reis de Ávila G., Castro K. L., Guerra-Duarte C., Sanchez E. F., Nguyen C., Granier C., Molina F., Chávez-Olortegui C.. Vaccine.2016;34(14). CrossRef
- Self-adjuvanted mRNA vaccination in advanced prostate cancer patients: a first-in-man phase I/IIa study Kübler H, Scheel B, Gnad-Vogt U, Miller K, Schultze-Seemann W, Vom Dorp F, Parmiani G, Hampel C, Wedel S, Trojan L, Jocham D, Maurer T, Rippin G, Fotin-Mleczek M, Mülbe F, Probst J, Hoerr I, Kallen K, Lander T, Stenzl A. Journal for Immunotherapy of Cancer.2015;3. CrossRef
- World Health Organization. (2020). Africa. International Agency for Research on Cancer doi:https://gco.iarc.fr/today/data/factsheets/populations/903-africa-fact-sheets.pdf..
- World Health Organization. (2020). United States of America. International Agency for Research on Cancer doi:https://gco.iarc.fr/today/data/factsheets/populations/840-united-states-of-america-fact-sheets.pdf..
- EpCAM expression in primary tumour tissues and metastases: an immunohistochemical analysis Spizzo G, Fong D, Wurm M, Ensinger C, Obrist P, Hofer C, Mazzoleni G, Gastl G, Went P. Journal of Clinical Pathology.2011;64(5). CrossRef
- Intraperitoneal immunotherapy with T cells stably and transiently expressing anti-EpCAM CAR in xenograft models of peritoneal carcinomatosis Ang WX , Li Z, Chi Z, Du S, Chen C, Tay JCK , Toh HC , Connolly JE , Xu XH , Wang S. Oncotarget.2017;8(8). CrossRef
- Determinants of snakebite mortality in Asia: A systematic review Hannan Wan Ibadullah WA , Azmi MF , Abas MI , Syed Abdul Rahim SS , Jeffree MS , Azhar ZI , Hayati F, Hassan MR . Annals of Medicine and Surgery (2012).2021;62. CrossRef
- Influenza Viruses: Innate Immunity and mRNA Vaccines Lee S, Ryu J. Frontiers in Immunology.2021;12. CrossRef
License

This work is licensed under a Creative Commons Attribution-NonCommercial 4.0 International License.
Copyright
© Asian Pacific Journal of Cancer Biology , 2022
Author Details