Comparison of Different Dosimetric Indices for Volumetric Arc Modulated Treatment Planning Using Two Different Treatment Planning Systems: A Feasibility Study for Total Body Irradiation
Download
Abstract
Objective: To compare two different treatment planning systems (TPSs) for plan quality and to assess the feasibility of volumetric modulated arc therapy (VMAT) for total body irradiation (TBI) on the Elekta Versa HD linear accelerator.
Materials and Methods: Ten clinical VMAT TBI plans were replanned in Monaco TPS version 5 for retrospective dosimetric analysis and to evaluate the feasibility of treatment delivery on the Elekta Versa HD. A dose of 12 Gy in six fractions was prescribed to cover 95% of the planning target volume (PTV). Organs at risk (OARs) included the lungs, kidneys, heart, liver, and brain. Dosimetric optimization and calculations were performed in Monaco TPS with a statistical uncertainty of 1% and a 2 mm grid. Plan quality was assessed based on dosimetric indices such as conformity and homogeneity index, as well as OAR doses. Comparisons of optimization time, monitor units, and beam-on time were also analyzed. Pre-treatment verification was conducted using an Octavius phantom, and gamma analysis was performed using Low's method with a dose difference of 3% and a distance to agreement of 3 mm criteria. The dosimetry of two VMAT TBI plans was compared using the Wilcoxon signed-rank test.
Result: The calculated average maximum and mean doses to the PTV were 14.3321 Gy and 12.235 Gy for Eclipse, and 14.428 Gy and 12.198 Gy for the Monaco system, respectively. Planning time in Monaco was approximately 4.5-6 hours compared to 14-18 hours for Eclipse TPS. Statistically significant differences were observed between the results of the two TPSs in terms of monitor units, mean doses to the lungs, kidneys, heart, and liver, and planning time (P < 0.05). However, calculated p-values for homogeneity index (HI), conformity index (CI), and brain dose for both VMAT TBI plans were not statistically different.
Conclusion: This study demonstrated the feasibility of VMAT TBI for the VERSA HD, offering faster and better optimization for a single target compared to Eclipse.
Introduction
Total body irradiation (TBI) is a radiation technique that is frequently used as a conditioning regimen for allogeneic hematopoietic stem cell transplantation (HSCT) or bone marrow transplantation (BMT) with chemotherapy [1]. The first study which showed that “stem cells” could recapitulate the blood system was reported by Till and McCulloch in the 1960s [2]. TBI used in conjunction with chemotherapeutic agents has proven to be useful for eradicating malignant cells from the blood formation sites. It also performs an immune-suppressive role by preventing rejection of donor hematopoietic cells, aiding successfully BMT, and leading to superior treatment outcomes for HSCT [3-4]. TBI was developed to irradiate the whole body uniformly with a prescribed dose while minimizing radiation induced complications mainly to the lungs and kidneys. A dose uniformity of ±10% was accepted in TBI [1, 5]. Initially, TBI was given with parallel opposed field or extended source skin distances (SSD) or other conventional methods which had their limitation like fabrication, a time-consuming process in addition to acute and late toxicities as sparing of OARs was difficult [6]. Also, setup errors of a few millimeters in the longitudinal direction at the junction volume can produce dose inhomogeneity [7]. The feathering technique can help to reduce dose inhomogeneity in which the longitudinal location of the junction is varied across treatment fractions [8]. But this process was cumbersome, time-consuming, and required multiple setups. To overcome these difficulties of traditional treatment techniques, many new systems are available in which overlapping fields/arcs can be used at different iso-centers. Gradient dose-based optimization (GDO) technique use gradually decreasing/increasing junction volumes and base/bias dose option through inverse planning to deliver homogeneous dose with better sparing of OARs. Tomotherapy based VMAT TBI allows better OAR’s sparing along with good homogeneous dose coverage of the target [9]. However, the attempt to deliver a clinically acceptable plan to the whole body only results in complicated planning which is mainly dependent upon the particular available treatment planning system (TPS) and dose delivery methods. Eclipse uses the PRO 3 optimization and AAA for dose calculation algorithm while Monaco is a Monte Carlo (MC) based algorithm, which uses 3 beam model components: transmission probability filters (TPFs), virtual source model (VSM), and X-ray voxel MC engine to achieve dose calculation accuracy. Monaco performs 2 stage optimizations: fast pencil beam for ideal fluence generation/distribution and the segmentation process includes segment shape, and segment weight for generating the best possible clinically deliverable VMAT TBI plans.
The aim of this retrospective study is to compare dosimetric quality and competency/efficacy of VMAT TBI plans generated on 2 different TPSs, 2 different delivery modalities using the same CT data sets in head-first supine position and to check for the feasibility of VMAT TBI on newly installed Versa HD linear accelerator in our institution.
Materials and Methods
1.1. Patient selection, contouring and dose prescription
Upper and lower body treatment planning computed topographic images with 5mm slice thickness of 10 selected patients were transferred to the Monaco TPS through Digital Imaging and Communication in Medicine (DICOM) with all delineated structure sets. All selected patients had already completed treatment on the Varian trilogy and pre-treatment QA was performed using the Artemis-Rapid Arc/VMAT QA Module of EPIQA [10]. To perform a meaningful evaluation and comparison, delineated PTV was given a 5mm inner margin after subtracting OARs. The included OARs for both the TPSs planning were brain, bilateral lungs, heart, liver, and kidneys. A single PTV optimization was performed in the Eclipse TPS. PTV was divided into three-four sub-PTVs as per the length of treatment to generate a clinically deliverable plan [11]. The bilateral lungs used for optimization were given a 2mm inner margin due to their proximity to the PTV and to deliver adequate dose to marrow in the ribs. This may reduce conflicts to the optimizer attempting 2 different optimization parameters in adjacent contours. The prescribed dose to target volume was 12Gy/6#, 2# daily six hours apart. The objective was to cover 95% of the target with 90% of the prescribed dose. The PTV and other included OARs for each selected patient are shown in Table 1.
Target Volume | Prescription Dose |
PTV (up to mid-thigh) | 12Gy/6#: 2Gy/# daily 2# 6 hourly apart |
Lungs, Kidneys, Heart, Liver, & Brain | < 10Gy |
PTV, planning target volume after subtracting both lungs and kidneys, liver, heart, and brain; B/L: bilateral
The mean dose constraints for OARs were ≤ 10 Gy.
1.2. Planning Process and plan objectives
The Eclipse TPS-generated plans were used to deliver treatment in a Varian Trilogy linear accelerator. CT data sets from the Eclipse TPS through DICOM were exported to Elekta Monaco TPS version 5.11.03 and these were used to generate VMAT TBI plans for dosimetric verification on the VERSA HD unit. Eclipse uses Progressive Resolution Optimizer 3 (PRO3) for optimization and Anisotropic Analytical Algorithm (AAA) to calculate dose distribution from external photons. Both TPSs had different optimization and dose calculation algorithms. The Eclipse system accounts for tissue heterogeneity anisotropically in the entire three-dimension of interaction site and increases the accuracy of scattered dose calculation. The AAA has 2 components: a configuration algorithm and an actual dose calculation algorithm. But Monaco offers constrained optimization, which means the optimizer varies the plan to optimize the dose corresponding to user set objectives (i.e., dose to the target volume) while meeting OAR’s hard constraints specified by the planner. Thus, the same planning parameters were chosen by fixing the same number and location of iso-centers, same field arrangement/overlapping junctions, longitudinal shifts, and same dose constraints. The same parameters used in both the plans in the study provided a meaningful and best possible dosimetric comparison. The dose delivery methods were also same in both the TPSs with static couch, moving gantry except collimation to the collimator head in Monaco.
1.2.1 Eclipse Planning
Treatment plan for the VMAT TBI was done using the Eclipse TPS version 11.0.31from Varian to deliver on Varian Trilogy equipped with Millennium 60 pair multileaf collimators (MLCs). The outer 20 pairs MLCs have a 1 cm projection at the iso-center and the inner 40 pairs are projected 0.5 cm at the iso-center. The whole PTV was optimized with 2 pairs of arcs each at 4 different iso-centers. Multiple plans for the eclipse were made to achieve a deliverable plan. Different arcs were arranged to have an overlap region of 3 cm on the superior/inferior beam edges and one open field arc at the chest and abdomen iso-center to get a uniform dose distribution. The optimization and dose calculation time for a single PTV was approximately 6-8 hrs. The system also has a maximum limit of 10 arcs in one plan. Initially, for treatment delivery on eclipse PTV was divided into 4 different sub-PTVs to optimize for each PTV individually, in less time, and it also helped to avoid the maximum limit of arcs. Two arcs per iso-center clockwise (CW) and counter-clockwise (CCW) with one additional arc at each iso-center with collimator rotation of 900 were used. The base dose tool was used to optimize different PTVs and smoothen doses at different arc junctions. After comparing the plan with four-different target plans, a good homogeneous deliverable plan was generated. The plan sum was the total plan consisting of arcs for different PTVs.
Monaco Planning TPS version 5.11.03 was used to create VMAT TBI plans for 10 selected patients. Monaco TPS employs the Monte Carlo (MC) algorithm, which is considered the most accurate algorithm available. The single PTV optimization was performed in 2 stages. The first stage used a fast Pencil Beam algorithm (PBC) to generate an ideal fluence distribution and the second stage optimized the shape and weight of the segment, so that a clinically deliverable plan could be generated.
2.2 VMAT TBI plan analysis, comparison, and verification
The clinically deliverable plans generated on both Eclipse and Monaco was normalized to deliver a 90% uniform dose to 95% of the target volume by subtracting the brain, the lungs, and bilateral kidneys. The field arrangement and overlapping region are shown in Figure 1 (a-c).
Figure 1. (a and b). Beam Isocenters in Coronal and Sagittal Plane. 1(c) Displaying Isocenter and Overlapping Region.
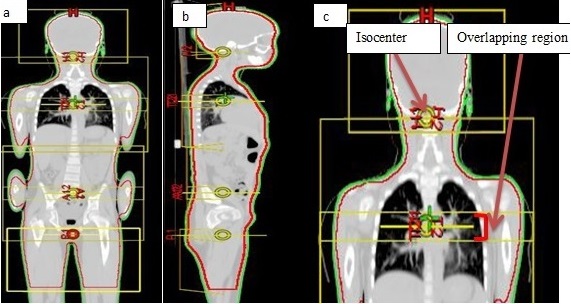
VMAT TBI plans made on Monaco were analyzed and compared with plans generated on eclipse. PTV mean and maximum doses, doses delivered to 95% of target, body maximum dose, and OAR mean doses were compared. Homogeneity index (HI) was calculated using D2% and D98% dose and D50% dose-volume histogram parameters and conformity index was calculated as per ICRU 83 definition [12]. The used formalism is:
HI = D2 % -D98 % / D50 %
An HI of zero indicates that the absorbed-dose distribution is almost homogeneous. Analysis of total MU’s, planning, and dose delivery time was also done. Verification plans were created for both TPSs in already acquired PTW Octavious phantom large image data sets (50cm) and verified in synchronized rotational gantry angles using an inclinometer. The 2 measurements were taken for the phantom in face-in and face-out positions and composed together to find uniformity of doses at the different arc junctions and within arc fields. The gamma evaluations for quality assurance (QA) were done using Low’s method for a dose difference between 3% and a distance agreement of 3 mm.
Results
Both TPS plans were compared for PTV coverage, OAR sparing, optimization and dose calculation time, MU’s to be delivered, and beam on time. The dose coverage in different planes for both TPS plans is shown in Figure 2.
Figure 2. Dose Distribution in Axial, Coronal, and Sagittal Planes for Eclipse and Monaco.
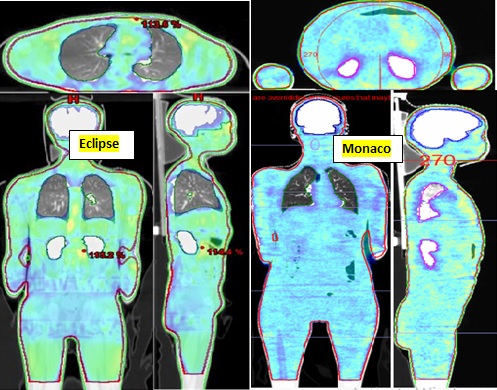
The axial, coronal, and sagittal planes represent homogeneous dose distribution, proper dose sculpting, and OARs sparing in both TPSs.
1.1 PTV analysis
The Monaco TPS VMAT TBI plans resulted in good dose coverage of PTV than eclipse plans. The average maximum and mean doses of planning target volume were 14.3827Gy±0.389Gy and 12.235Gy for the Eclipse; and 14.535Gy±0.164Gy and 12.198Gy for the Monaco system, respectively. HI for the PTV was comparable in both TPSs (Table 2).
PTV | Eclipse TPS | Monaco TPS | P |
HI | 0.22±0.03 | 0.18±0.03 | 0.051 |
CI95% | 0.91± 0.02 | 0.91± 0.02 | 0.03 |
MU | 2082.3±473.9 | 6681.4±1210.2 | 0.00000006 |
HI, homogeneity index; CI conformity index; MU, monitor unit; †Wilcoxin test P-value<0.05
The CI was better in Monaco (p < 0.05). The comparative DVH for PTV for the two TPS plans is shown in Figure 3a.
Figure 3. a) DVH for PTV in two TPSs; b) DVH for OARs in two TPSs.
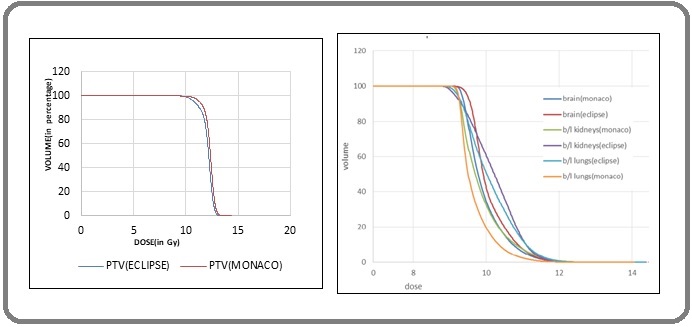
The variation of HI and CI for both TPSs was also plotted and is shown in Figures 4 (a and b).
Figure 4. HI & CI Variation, E & M Represents Eclipse & Monaco, Respectively.
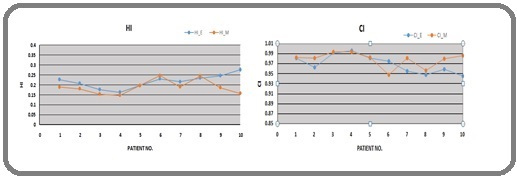
1.2 OAR doses
The comparison of average mean doses received by the lungs, kidneys, liver, heart, and brain are shown in Table 3; a comparative DVH for OARs is shown in Figure 3b.
OARs (Avg_mean) | Monaco TPS (Avg_mean±SD) | Eclipse TPS (Avg_mean±SD) | P |
Brain | 10.105±0.4273 | 10.113±0.0474 | 0.954 |
Lungs | 9.9724±0.3498 | 10.230±0.0634 | 0.049 |
Kidneys | 9.9214±0.2672 | 10.252±0.1167 | 0.01 |
Liver | 9.9883+0.062 | 10.1573+0.1206 | 0.00745 |
Heart | 9.9403+0.1246 | 10.0775+0.1163 | 0.00436 |
†Wilcoxin test P-value<0.05
The mean dose in the brain was comparable in the 2 TPS plans. The mean doses for lungs, kidneys, liver, and heart were higher for the Eclipse than Monaco, which was statistically significant (p < 0.05) and shown in Figure 5.
Figure 5. Mean Dose Difference of OARs between two TPSs.
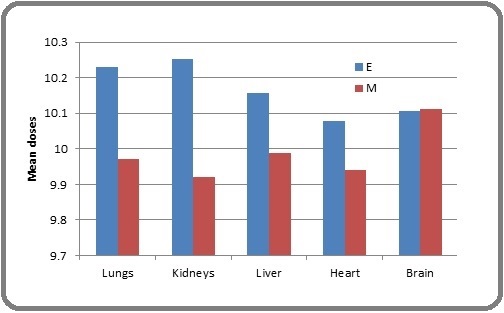
1.3 Monitor units, planning time and time for delivery
The total MUs for all arcs to be delivered were 2082.3 ± 473.9, and 6681.4 ± 1210.2, respectively for the Eclipse and the Monaco TPS (Table 2 and 4).
Pt. no. | 1 | 2 | 3 | 4 | 5 | 6 | 7 | 8 | 9 | 10 | Mean ± SD |
MU_E | 1814 | 2095 | 1872 | 2176 | 1879 | 2014 | 1567 | 1851 | 3309 | 2246 | 2082.3±473.9 |
MU_M | 6530 | 7250 | 4942 | 5813 | 6384 | 7869 | 5178 | 6743 | 8929 | 7174 | 6681.2±1210.3 |
MU_E, monitor unit in Eclipse; MU_M, monitor unit in Monaco
For each iso-center, MUs were 694.13 ± 157.97 and 22273±403.4 for the Eclipse and the Monaco plans, respectively. The Monaco plans MUs were approx. 3.2 times more than the Eclipse TPS plans (Table 2). The average time to deliver a VMAT TBI plan in Versa HD was approximately 14–18 min but Varian trilogy took around 18-22 minutes.
Plan Verification
The plan verification was performed using Octavious phantom. The average gamma passing rate was 97.2% for the Eclipse and 97.6% for Monaco. Figure 6 shows a screenshot of the composed plan in the abdomen region.
Figure 6. Screenshot Showing Volume Analysis, Gamma, and Dose Distribution Agreement.
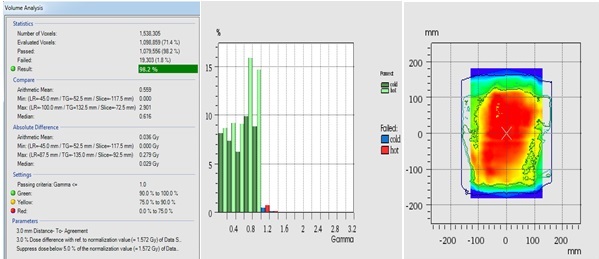
On 3D volume analysis, an average gamma was 98.1% and 97.8% for the Eclipse and Monaco, respectively. Figure 6 is displaying the average gamma passing point for the 3%, 3mm criterion.
Discussion
Total body irradiation is a useful conditioning regimen for patients undergoing BMT for hematological malignancies. In this study, we compared 2 different TPSs for plan quality and competency of VMAT TBI on the Elekta linear accelerator ‘Versa HD’. The average maximum dose in Monaco was 15.23cGy more than the Eclipse and the observed difference in average mean doses was 3.70 cGy which was not significantly different. The mean lung and kidney, liver, and heart doses were significantly higher for the Eclipse than Monaco but brain doses were similar. In this study, OAR doses were more than the mean lung dose threshold reported by Volpe et. al 9.8Gy versus 9.4Gy [8]. Our objective was to restrict the mean doses of the OARs to ≤10 Gy. From the HI and CI obtained in the current study, we can conclude that both the TPSs had the potential to create clinically deliverable plans with a good sparing of OARs. Our results was similar to those reported by Lu W., Adrian N., and Aleksi [11, 13-15]. Planning and dose calculation time was three times less for Monaco as compared to Eclipse. But a significant beam on time difference (2-4 minutes) was observed because MU’s delivered were three times more in Monaco (Table 4 and 5).
Parameters compared | Monaco TPS | Eclipse TPS |
Optimization and Dose Calculation time | * 4.5 – 6 hrs. | * 21-27 hrs. |
Monitor Units | ** 6681.2±1210.3 | ** 2082.3±473.9 |
Set Up Positioning and Delivery time | 1.2 hrs. first day & 35-40 min. rest treatment | 1.2 hrs. first day & 40-44 min. rest treatment |
*two optimization, ** may be due to algorithm difference
The more number of MUs is the result of algorithm difference of TPSs. The benefits of TBI are well published and documented [16]. In the presented study both TPSs produced comparable dose distribution in term of HI and CI.
A single PTV optimization in the Monaco TPS offers benefits for the planner rather than optimizing sub-PTVs in the Eclipse TPS for clinically acceptable plan generation. Although Eclipse TPS offers single PTV optimization it takes a longer time to complete optimization. Sometimes overlapping OARs in sub-PTVs create a conflict in achieving dose constraint. In a study, Springer et al. showed satisfactory dose distribution in the whole-body target for linac-based VMAT TBI in 7 patients using 9-15 iso-centers [17]. This large field planning of VMAT TBI is very complex due to multi-isocentric overlapping arcs. So necessary quality assurance tests are needed using an appropriate/ available phantom. In our study, we used 3–4 iso-centers with a 3cm overlap region. The dose distribution in the critical junction at the thigh region was controlled using base/bias dose properties in both the TPSs. The delivered doses were monitored to ensure that they were quite close to the prescribed dose, and this helped in dose adjustment of the fractional dose if needed. In our study, we used an ionization chamber, gaf-chromic film, and diode to monitor the delivered dose. In a study, Springer et al. used MOSFET for measurements, and reported results were within 5% accuracy [17]. In another study, Ganapathy K et. al used the TLD-100 LiF and found that the dose homogeneity was within + 10% [18]. Various phantoms and detectors are available for dosimetric verification and any of them can be used for verification. Hui et. al. performed plan verification in their study using a cylindrical phantom [19]. Zhuang et. al. used a solid water phantom of 30 cm x 30cm x 12cm with gaf-chromic film and a 0.6cc farmer chamber in their study [20]. None of the available systems are efficient to verify large fields. In this study, we used an Octavious phantom, which could measure a dose up to 50cm in length. Two measurements were taken, first in face-in and second in face-out position. Both measurements were composed together to find dose uniformity along the entire length of arcs and the junction of 2 adjacent isocentric arcs. Before verifying the VMAT plan with Octavious phantom, doses were verified with gafchromic films of 5x15cm2 along the overlapping region (the study is in progress and not in the scope of the current study). Our results are also comparable to those of Zhuang et. al, and Srivastava et.al where it was concluded that gamma analysis (3%, 3mm) with a 10% dose threshold means a good dose comparison between generated and delivered doses [20, 21].
Hence in conclusion we can conclude that both the Eclipse and the Monaco TPSs were capable of a high degree of target dose conformity and critical organ sparing therefore either of the TPS systems can be used for VMAT TBI plan generation. But the planning of VMAT TBI using Monaco is easier as it offers faster optimization on a single target compared to the Eclipse, where sometimes a larger length target is split into 3 to four segments to get a better-quality plan. The MC-based dose calculation accuracy with robust optimization tools in Monaco allowed a much faster generation of VMAT TBI plans. The plan analysis and dosimetric comparison showed the feasibility of VMAT TBI treatment using the Monaco planning software on Versa HD.
The limitations of our study are that it was a dosimetric study, so no clinical information could be derived from our results. The plan analysis and dosimetric comparison showed the feasibility of VMAT TBI treatment using the Monaco planning software on Versa HD. An elaborative planning clinical study is in progress to assess the further scope for improved analysis.
Acknowledgements
General
We would like to thanks all patients’ whom data was used for this retrospective analysis and made us capable to utilize our versa HD for VMAT TBI treatments. Also I would like to thank Head, Radiation Oncology Department for the free access to work.
Funding Statement
No funds involved.
Approval
Yes, approved by the institute collaborative committee.
Conflict of Interest
No conflicts of interest.
Ethical Declaration
Yes, approved by institute ethical committee.
Authors Contribution
Reena Sharma: conceptual design, treatment planning, data collection, analysis, manuscript writing and approval. Dr. Budhi Singh Yadav: conceptual design, patient selection, treatment planning approval, manuscript writing and approval.
Dr. Pankaj Kumar: conceptual design, manuscript writing and approval.
Data Availability
The data that support the finding of this retrospective analysis is available from corresponding author upon reasonable request.
References
- The Physics of Radiation Therapy G Khan FM , Gibbons JP . Philadelphia: Lippincott Williams & Wilkins. 2014; 5th ed..
- A direct measurement of the radiation sensitivity of normal mouse bone marrow cells Till JE , Mcculloch EA . Radiation Research.1961;14.
- Factors associated with pulmonary toxicity after myeloablative conditioning using fractionated total body irradiation Byun HL , Yoon HJ , Cho J, Kim HJ , Min YH , Lyu CJ , Cheong JW , et al . Radiation Oncology Journal.2017;35(3). CrossRef
- Total Body Irradiation: Guidelines from the International Lymphoma Radiation Oncology Group (ILROG) Wong JYC , Filippi AR , Dabaja BS , Yahalom J, Specht L. International Journal of Radiation Oncology, Biology, Physics.2018;101(3). CrossRef
- Physical aspects of total and half body photon irradiation Van DJ , Galvin JM , Glasgow GP , Podgorsak EB . AAPM Report No.1986;17.
- Whole body radiotherapy: A TBI-guideline Quast U. Journal of Medical Physics / Association of Medical Physicists of India.2006;31(1). CrossRef
- Junctioning longitudinally adjacent PTVs with Helical TomoTherapy Garcia LM , Gerig LH , Raaphorst P, Wilkins D. Journal of Applied Clinical Medical Physics.2010;11(2). CrossRef
- Lethal pulmonary complications significantly correlate with individually assessed mean lung dose in patients with hematologic malignancies treated with total body irradiation Della Volpe A, Ferreri AJM , Annaloro C, Mangili P, Rosso A, Calandrino R, Villa E, Lambertenghi-Deliliers G, Fiorino C. International Journal of Radiation Oncology, Biology, Physics.2002;52(2). CrossRef
- Clinical feasibility of TBI with helical tomotherapy Peñagarícano JA , Chao M, Van Rhee F, Moros EG , Corry PM , Ratanatharathorn V. Bone Marrow Transplantation.2011;46(7). CrossRef
- EPIQA: A robust tool for the quality assurance of total body delivery Reena K, Pankaj K, Arun OS . AIP Conference Proceedings.2019;:140020-1-4.
- A non-voxel based broad beam (NVBB) framework for IMRT treatment planning. Phys Lu W. Med Biol.2010;55:7175-7210.
- Report 83 Oxford University Press “The International Commission on Radiation Units and Measurements Journal of the ICRU.2010;10(1).
- Dosimetric comparison of helical tomotherapy treatment plans for total marrow irradiation created using GPU and CPU dose calculation engines Nalichowski A, Burmeister J. Medical Physics.2013;40(7). CrossRef
- Dosimetric evaluation of total marrow irradiation using 2 different planning systems Nalichowski A, Eagle DG , Burmeister J. Medical Dosimetry: Official Journal of the American Association of Medical Dosimetrists.2016;41(3). CrossRef
- Analytical Anisotropic Algorithm Calculation in Total Body Irradiation: A Comparison with Monte Carlo Calculation and Dosimetry Aleksi S, Jarkko O, Jani K. Cancer Sci Clin Ther.2021;5(4):532-547.
- Is there a better way to deliver total body irradiation? Appelbaum FR , Badger CC , Bernstein ID , Buckner CD , Deeg HJ , Eary JF , Matthews DC , et al . Bone Marrow Transplantation.1992;10 Suppl 1.
- Total body irradiation with volumetric modulated arc therapy: Dosimetric data and first clinical experience Springer A, Hammer J, Winkler E, Track C, Huppert R, Böhm A, Kasparu H, et al . Radiation Oncology (London, England).2016;11. CrossRef
- Patient dose analysis in total body irradiation through in vivo dosimetry Ganapathy K, Kurup PGG , Murali V, Muthukumaran M, Bhuvaneshwari N, Velmurugan J. Journal of Medical Physics.2012;37(4). CrossRef
- Helical tomotherapy targeting total bone marrow - first clinical experience at the University of Minnesota Hui SK , Verneris MR , Higgins P, Gerbi B, Weigel B, Baker SK , Fraser C, Tomblyn M, Dusenbery K. Acta Oncologica (Stockholm, Sweden).2007;46(2). CrossRef
- Dosimetric study and verification of total body irradiation using helical tomotherapy and its comparison to extended SSD technique Zhuang AH , Liu A, Schultheiss TE , Wong JYC . Medical Dosimetry: Official Journal of the American Association of Medical Dosimetrists.2010;35(4). CrossRef
- Gamma Evaluation with Octavius 4D Phantom for Pre-Treatment of Modern Radiotherapy Treatment Techniques Srivastava RP , Basta K, Thevissen K, Junius S, Vandeputte K, Wagter CD . Int J Nuclear Med.2019;2(2):000117.
License

This work is licensed under a Creative Commons Attribution-NonCommercial 4.0 International License.
Copyright
© Asian Pacific Journal of Cancer Care , 2023
Author Details
How to Cite
- Abstract viewed - 0 times
- PDF (FULL TEXT) downloaded - 0 times
- XML downloaded - 0 times