Breast Cancer Therapies: A Review
Download
Abstract
Breast cancer is the most frequent cause of cancer-related deaths among postmenopausal women. It is the leading cause of cancer-related death in women worldwide. The human epidermal growth factor receptor 2 (HER2) is overexpressed in roughly 30% of all breast cancers. Breast cancers that are human epidermal growth factor receptor 2 (HER2) -positive are frequently and especially aggressive, as this receptor has been shown to promote cancer growth. Before the advent of HER2-specific monoclonal antibodies, HER2-positive breast cancers had a poor prognosis. Clinical results for HER2-positive breast cancer have, however, changed significantly since the introduction of monoclonal antibodies and antibody-drug conjugates in therapy. Monoclonal antibodies (mAb) such as trastuzumab and pertuzumab attach to the HER2 receptor protein, deactivating it or mobilizing the immune system to aid in the destruction of cells that produce it. The remarkable accomplishments in the development of HER2-targeted therapies, along with a better understanding of the disease’s biology, have improved clinical outcomes over the years. Resistance however still poses a significant challenge, emphasizing the vital need of developing novel agents. This review article examines the mechanisms underlying the action of these therapies with an overview of their advancements and setbacks in breast cancer treatment in the last decade.
Introduction
The World Health Organization (WHO) estimates that about 2.3 million women were diagnosed with breast cancer in 2020 with 685 000 global deaths. This estimation classifies breast cancer as the most common type of cancer worldwide (12.5% of all cancers). Clinically, breast cancers can be classified based on immunohistochemical findings into luminal A, luminal B, basal-like, and (HER2) over-expressing. This classification is based on the receptors found on the surface of these malignant cells [1]. Luminal A and B type tumors have estrogen and progesterone receptors on their surface, tend to grow at a slower rate and have a good prognosis. Meanwhile, HER2-positive tumors frequently exhibit metastasis, higher proliferation rates, and less well-differentiated growth. About 20 to 30% of these cancers have HER2 amplification or overexpression [2]. As a result of this, overexpression of HER2 is associated with aggressive tumor behavior and a worse prognosis [3].
HER2, a 185 kDa protein (p185), is a member of the epidermal growth factor receptor (EGFR) family on account of its tyrosine kinase activity [4, 5]. EGFR/ HER1, HER2, HER3, and HER4, also known as ErbB1, ErbB2, ErbB3, and Erb4, respectively, are the four primary epidermal growth factors known in humans [5]. These receptors control cell proliferation, differentiation, and survival by binding growth factor ligands and forming dimers. The four HER receptors consist of a cysteine-rich extracellular component that binds ligands, a lipophilic trans-membrane segment, and an intracellular tyrosine kinase domain [6].
Among the four HER receptors, HER2 is the only one with no known naturally occurring high-affinity ligand. While the HER receptors can form homodimers or heterodimers on binding to ligands, HER2 preferentially forms heterodimers, with HER3 being the preferred partner [5, 6]. Despite HER2 lacking a natural ligand and HER3 lacking an intracellular tyrosine kinase activity, HER2/HER3 heterodimers are more stable and initiate signaling pathways that are more potent than other dimers. This highlights the role HER2 plays in the growth of tumor cells that overexpress this receptor [5-8].
The HER2 receptor has evolved into a crucial therapeutic target for the treatment of cancer for a number of reasons. First, tumorigenesis is caused by increased HER2 levels, and cancer cells express significantly more HER2 than healthy adult cells. Second, both original tumors and metastasized tissues exhibit HER2 overexpression. In addition, HER2 is the preferred dimerization partner for other HER receptors when associated signaling pathways are activated. Heterodimers including HER2 have the highest mitogenic potential of any HER complex. The phosphatidylinositol-3-kinase (PI3K) and mitogen-activated protein kinase (MAPK) pathways, which can lead to cancer, are among the intracellular signaling cascades that are prevented from being activated by inhibiting HER2 dimerization. Despite the fact that HER2 is overexpressed in a variety of malignancies, the majority of cancer therapies that target HER2 are explored in breast cancer [4].
Functional Mechanism of the HER2 Receptor
While the functional mechanism of the HER2 receptor is simple in organisms like Caenorhabditis elegans and Drosophila, it is far more complex in mammals, especially humans where at least twelve ligands and 4 receptors are required for signaling [9]. When HER2 receptors are bound to ligands, they undergo dimerization. This leads to the auto-phosphorylation of the tyrosine residues in the cytoplasmic portion of the receptor. Due to this conformational shift, the tyrosine kinase domain can now adopt an active conformation. Additionally, a large number of downstream signaling proteins with Src homology-2 (SH2) or phosphotyrosine-binding (PTB) domains are recruited via different pathways and activated by autophosphorylation of the HER receptors [10]. The most important pathways include the MAPK, PI3K and protein kinase C (PKC) pathways. These pathways begin with Ras, a self-inactivating signal transducer.
Protein kinase B (also known as AKT) is phosphorylated and activated as a result of PI3K pathway activation. This leads to the phosphorylation of several downstream targets. Decreased breakdown of cyclin D1 and transcription of p27 (a cyclin dependent kinase 2 inhibitor) are two additional downstream effects of the PI3K-AKT pathway [3]. Cyclin D1 is a protein required for cell cycle progression at the G1/S transition point. Glycogen synthase kinase 3 beta (GSK-3β) phosphorylates cyclin D1, thereby promoting its transport from the nucleus to the cytoplasm where it is degraded via the ubiquitin-proteasome pathway. AKT inhibits GSK-3β and in doing so stabilizes cyclin D1 [11]. P27 in high levels are also required to maintain quiescence in the cell. The accumulationcumulative effect exerted by the PI3K-AKT pathway eventually increases cell proliferation and survival [3, 11]. Figure 1 summarizes the HER family signaling pathway.
Figure 1. The Dimerization of the Human Epidermal Growth Factor Receptor (HER) Family Members (HER1/HER2, HER1/HER3, HER2/HER3, HER3/ HER4), Leads to the Autophosphorylation of these Receptors. These receptors then activate downstream protein kinases (PI3K/AKT, Ras/Raf/MAPK), that switch on various transcription processes, increasing cell survival, proliferation, and angiogenesis [12]. .
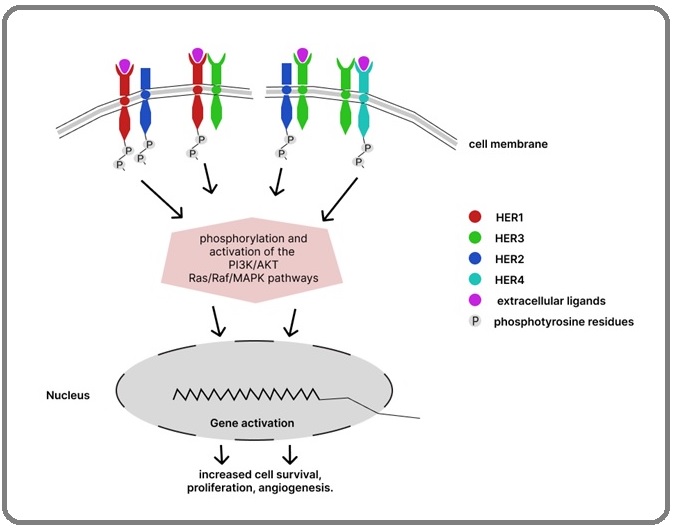
In breast tumor cell lines and cancers, the overexpression of HER2 also leads to the activation of the Ras/MAPK signaling pathway regulated by two main factors, MAP kinase kinase (MEK) and MAPK. These factors are required for the proliferation of cells. The pathway begins with Ras-activation of phospholipase C (PLC), leading to the activation of PKC. PKC, like other factors discussed, activates various transcription elements that lead to the expression of genes that affect cell growth and survival [3]. The HER receptors can also directly interact with transcription factors for genes like COX2, TP53, and CCND1 (Cyclin D1) via the nuclear localization pathway. These receptor/receptor complexes may be transported to the nucleus with their ligands, in spliced form or without any alteration [4].
Problems with Current Chemotherapy Treatments
Chemotherapy, immunotherapy, surgery and radiotherapy have traditionally been the cancer treatment pillars. Cancer immunotherapy did not exist until the late twentieth century. In the last ten years, cytotoxic chemotherapy in breast cancer has made significant progress, with several landmark studies identifying clear survival advantage for newer therapies. Personalized breast cancer therapies based on tumor molecular characteristics have improved the risk-benefit ratio of current therapies [13].
Breast cancer is still a classic model in which chemotherapy has been tested and shown to have tolerable toxicity. Trastuzumab, a humanized monoclonal antibody against HER2, is the first scientific proof of a rationally designed, specifically aimed biological breast cancer treatment. Trastuzumab, when given in combination, improves overall survival in patients with metastatic disease. The advent of trastuzumab into routine treatment has transformed the prospects for patients with HER2-positive breast cancer dramatically. Nonetheless, some issues remain with trastuzumab use, such as its role in advanced disease or low-risk disease, cardiovascular safety in older adults, and drug resistance [14, 15]. Figure 2 shows the chemical structure of Trastuzumab.
Figure 2. Chemical Structure of Trastuzumab with the chemical formula C6470H10012N1726O2013S42 and molar mass 145531.86 g·mol−1 [43] .
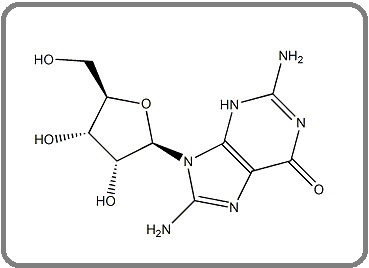
Despite the therapeutic advantage associated with the administration of trastuzumab, both de novo and acquired clinical resistance are now more widely acknowledged. Response rates to trastuzumab monotherapy range from 11 - 26%, which suggests that many tumors with HER2-amplified metastatic breast cancer would not respond to monotherapy. Additionally, the range of the trastuzumab-based therapeutic response time is from 5 to 9 months, indicating that acquired resistance frequently manifests [15, 16].
Recent research has shown that there is a significant correlation between trastuzumab resistance and mutational activation of the PI3K-AKT-mTOR signaling pathway, which promotes abnormal cell growth and proliferation in a number of tumor forms. Also, the buildup of p95-HER2, a shortened version of the HER2 receptor, has been suggested as another mechanism for mediating resistance to trastuzumab. A constitutively active kinase that can form dimers with other HER family members and trigger downstream signaling pathways is the amino-terminally truncated p95-HER2 [17].
How Breast Cancer Evades the Immune System
By orchestrating an immunosuppressive microenvironment and losing their immunogenicity, malignant cells can resist immune clearance. By tumor type and even by tumor lesion, a tumor’s ability to use these immune evasion pathways varies. Certain genes and pathways may be expressed or repressed in various malignancies, which results in a loss or reduction of antigenic expression, impaired immune cell function, and immunotherapy resistance. Cancer immunotherapy is fundamentally dependent on the immune system’s capacity to discriminate between benign and malignant cells, which partly depends on malignant cells maintaining an adequate level of antigenicity. By lowering their immunogenicity, tumors that would otherwise have enough antigenicity for immune detection can avoid being eliminated [16, 18].
For immune-mediated elimination to be effective, leukocyte infiltration into tumor tissue and identification of malignant cells are required. Cancer clones develop by a mechanism known as “cancer immunoediting” to evade immune-mediated destruction by leukocytes with anti-tumor characteristics. The recruitment of immunosuppressive leukocytes, which create a milieu that compromises the effectiveness of an anti-tumor immune response, may allow some cancers to evade removal. Therefore, clinically relevant tumors appear to be characterized by an immune system that actively selects for poorly immunogenic tumor clones and/or creates a microenvironment that inhibits productive anti-tumor immunity, despite the fact that the immune system can sometimes be used to combat tumors [16, 19].
Pathways Involved in Immune Checkpoint Inhibition
Under homeostatic conditions, a surfeit of immunosuppressive pathways exists to promote self-tolerance and protect against autoimmunity. Neoplasms have been shown to exploit these pathways thus preventing antitumor responses and gaining a survival advantage by escaping immune detection [20]. Immune checkpoint proteins are pivotal in maintaining the balance between auto-immunity and self-tolerance. Activation of immune checkpoints creates an immunosuppressive environment thus allowing the tumor cell to escape from immune-mediated obliteration [21]. Programmed cell death ligand 1 (PD-L1) expression is induced in vivo on human tumor cell lines and the expression of this checkpoint renders tumor-specific T cells inactive, thus serving as an immune escape mechanism in cancer immunotherapy. In breast cancer malignancies, PD-1/ PD-L1 inhibitor monotherapy in combination with chemotherapy has been reported to produce a positive outcome in early-phase trials [22, 23]. Certainly, inhibition of the relationship between PD-L1-expressing tumors and PD-1-expressing tumor-specific T cells via PD-1 or PD-L1 inhibiting antibodies accentuates the cytolytic effects of the T cells. The aim of immune checkpoint inhibitor (ICI) therapy is to intensify the anti-tumor immunity of the body by deranging the mechanisms that tumors use in evading the immune system. Immune checkpoint pathways in normal tissues, including cytotoxic T-lymphocyte antigen 4 (CTLA-4) and programmed cell death protein 1 (PD-1), down-regulate T-cell activity to prevent autoimmune diseases [24]. Figure 3 shows the mechanism of action of immunotherapeutic agents.
Figure 3. Chemical Structure of Lapatinib, with Chemical Formula C29 H26 ClFN4 O4 S and a molar mass of 581.06 g.mol−1 [44] .
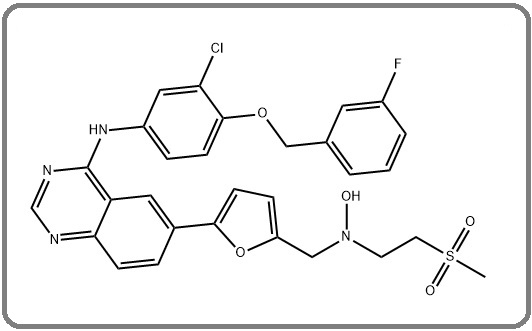
Current Immunotherapeutic Advances in Her2-Positive Breast Cancer Therapy
Cytotoxic T-lymphocyte antigen 4 (CTLA-4) Inhibitors.
Two monoclonal antibodies that inhibit CTLA-4 are currently either approved by The United States Food and Drug Administration (FDA) or under investigation.
Ipilimumab
Ipilimumab was the first immune checkpoint inhibitor to gain FDA approval in metastatic melanoma in 2011 [25]. It is a full human immunoglobulin IgG1 monoclonal antibody targeting CTLA-4 that has been approved both as monotherapy and in combination with nivolumab. Ipilimumab is a fully humanized IgG1 monoclonal antibody that blocks cytotoxic T-lymphocyte antigen 4 (CTLA-4). Blocking CTLA-4 removes an inhibitory signal from reducing the activity of T lymphocytes.
Tremelimumab
Tremelimumab is a human IgG2 monoclonal antibody directed against CTLA-4. It has a similar mechanism of action to ipilimumab, blocking the interaction between CTLA-4 and CD28, thus preventing CTLA-4-mediated immune-cell inactivation. It is currently being examined in combination with other immunomodulatory agents to determine whether it has clinical benefits in other cancer types [25].
PD-1/PD-L1 Inhibitors
Among others, Nivolumab and pembrolizumab are primarily clinically approved PD-1 inhibitors. Nivolumab and pembrolizumab have vastly altered the treatment of many advanced and metastatic cancer types. These treatments appear to show durable effects in a wide variety of malignancies, and the number of clinical trials being conducted with these agents is staggering. Nivolumab and pembrolizumab are IgG4 antibodies that bind to PD-1 with high affinity and have a low affinity for complement and Fc receptors [26]. This minimizes the host effector cell/immune engagement function of these antibodies, ensuring that they elicit their effects primarily by inhibiting PD-1–PD-L1 interactions by direct occupancy and steric blockade of the PD-L1 binding site of PD-1.
Pembrolizumab
It is established that the PD-1 pathway is frequently co-opted by tumors to evade an immune response. Pembrolizumab is a high-affinity, highly selective, humanized monoclonal IgG4-K antibody against PD-1 that provides dual ligand blockade of PDL1 andPD-L2. PD-L1 is not detected in normal breast tissue but is expressed in approximately half of all breast cancers, with expression generally higher in triple-negative breast cancers (TNBC) [27].
In the treatment of patients with high-risk early- stage TNBC, it is usually used in combination with chemotherapy as a neoadjuvant treatment followed by use as a single adjuvant agent following surgery. It is also used in combination with chemotherapy for the treatment of locally recurrent, unresectable or metastatic TNBC expressing PD-L1.
Atezolizumab
Atezolizumab is a humanized monoclonal antibody used to prevent the interaction of PD-L1 and PD-1, removing the inhibition of immune responses seen in many cancers. This medication is reserved for patients whose tumors express PD-L1, cannot receive platinum-based chemotherapy, or whose tumors do not respond to platinum-based chemotherapy.
Tki Advances in Her2+ Breast Cancers
In the past, general systemic chemotherapeutic drugs were often used to treat cancer, but these drugs have a pressing disadvantage, as they do not differentiate between normal cells and cancerous cells. However, translational medicine provides a new alternative [28]. By targeting HER2 in HER2+ breast cancers, clinicians can administer drugs with specificity for this growth factor [28]. Several of these drugs have been discovered, and while there is a growing problem of drug resistance relating to the first few developed drugs (trastuzumab and lapatinib), there continues to be research into better drugs to combat the ever-growing plight of breast cancer patients [29].
Lapatinib
Lapatinib, an oral EGFR/HER2 tyrosine kinase inhibitor (TKI), is the second anti-HER2 drug approved by the FDA [29-31].
Unlike the trastuzumab, lapatinib competes with adenosine triphosphate (ATP) for the ATP-binding domain of the tyrosine kinase receptor. This inhibits tyrosine kinase phosphorylation and consequently disrupts the downstream signaling pathways, MAPK and PI3K-Akt [31, 32]. One of the improvements of trastuzumab over lapatinib is its affinity for p95HER-2 as well [31]. This has also been demonstrated in mice and humans that when treated with lapatinib, tumors resistant trastuzumab were negatively affected [32]. Lapatinib has also been shown to halt tumor progression in HER2+ breast cancer patients when used as a first-line drug in treatment [30]. A combination of lapatinib with trastuzumab has proven to provide a pathological complete response when compared with a combination of lapatinib and taxanes in the NeoALTTO trial [33]. Lapatinib plus capecitabine is superior to capecitabine alone in women with HER2- positive advanced breast cancer that has progressed after treatment with regimens that included an anthracycline, a taxane, and trastuzumab. Lapatinib in combination with capecitabine has also been shown to reduce cancer progression drastically when compared to capecitabine alone, although the combination also proved to be more toxic [29].
There have been several adverse effects of lapatinib when used in clinical settings, ranging from diarrhea to pruritis to nausea. Fortunately, these adverse effects are mild, and lapatinib is mostly well-tolerated [30]. The more pressing issues with lapatinib relate to the growing problem of resistance. Resistance to lapatinib does not depend on the same factors that cause trastuzumab resistance, for example, the presence of p95HER2. However, it has been postulated that the use of lapatinib results in the overexpression of the estrogen receptor (ER) and cross-signaling. A study revealed that when lapatinib-resistant cell lines were compared with lapatinib-sensitive cell lines, the transcriptional activity of ER-associated signaling genes was higher in the lapatinib-resistant cell lines [31, 32]. Another proposed mechanism is the activation of the PI3K–Akt pathway, which is also one of the mechanisms of trastuzumab resistance [32].
Neratinib
Neratinib is asis an orally active TKI that irreversibly inhibits EGFR and HER2 receptors [29, 30] to bind through binding to the cysteine residues on the ATP-binding pockets.
Neratinib has been shown to delay the onset of brain metastases in patients with HER2+ breast cancers, in combination with paclitaxel [34]. When compared with trastuzumab and paclitaxel, it has been shown to be more beneficial [35]. In the phase II neratinib trial, neratinib was shown to facilitate a higher Overall Response Rate (ORR) in patients who have not been treated with trastuzumab, when compared with patients who had been pre-treated (56% versus 26%) [36]. The ORR was also slightly higher (64%) in another clinical study that compared lapatinib-naïve patients to lapatinib-treated patients (57%) [37]. The adverse effects common in these studies were diarrhea, nausea, and vomiting [29, 38]. Diarrhea is postulated to be caused by the inhibition of the EGFR receptor.
The NALA trial was a pIII trial that took place from 2013 through 2017, comparing the efficacy of neratinib plus capecitabine versus lapatinib plus capecitabine [35,39]. The study proved that neratinib plus capecitabine was the better drug combination, as this reduced the time for progression-free survival when compared to the lapatinib plus capecitabine combination. The patients with the neratinib plus capecitabine drug combination also required fewer interventions for CNS metastases, leading to the hypothesis that the drug combination delayed brain metastases. On the other hand, the ORR remained the same, and neratinib failed to improve the overall survival rate. These results culminated in the FDA approving the combination of neratinib and capecitabine for patients with HER2+ metastatic breast cancers (Figure 4) [35, 38, 39].
Figure 4. Chemical Structure of Neratinib, Chemical Formula C30H29ClN6 O3 , and Molar Mass 557.05 g·mol-1 [45] .
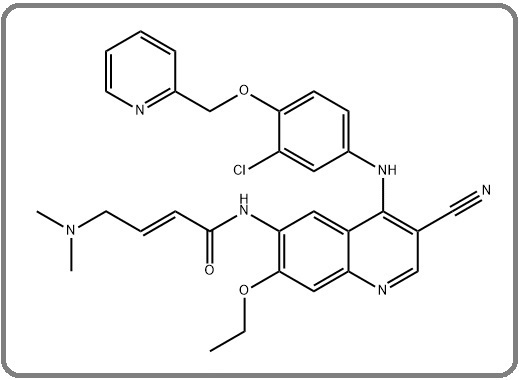
Pyrotinib
Pyrotinib is a second-generation, irreversible pan-HER TKI that targets the EGFR, HER2, and HER4 receptors (Figure 5) [39, 40].
Figure 5. The Chemical Structure of Pyrotinib with Chemical Formula C40H39ClN6O11and molar mass 815.22426 [46] .
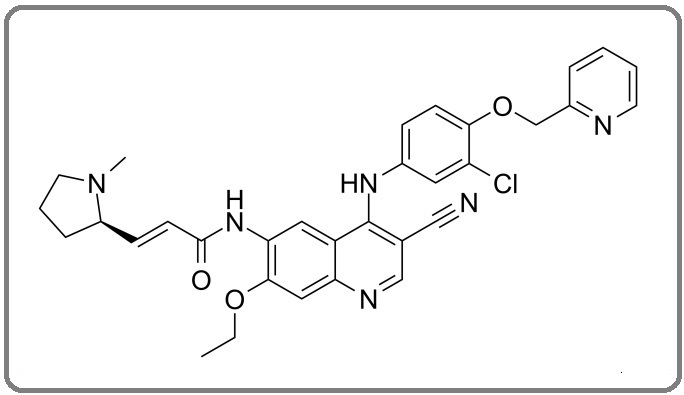
In a Phase II trial, the combination of pyrotinib and capecitabine was shown to be more effective than lapatinib and capecitabine, as the former had a higher objective response rate (78.5% versus 57.1%) and higher progression-free survival [41]. The PHOEBE trial, a Phase III trial conducted in China, also revealed that the combination of pyrotinib and capecitabine produced a higher number of patients with complete pathological response and a higher progression-free survival (PFS) rate when used in patients with HER2+ metastatic breast cancers, as compared with lapatinib and capecitabine [42]. It was postulated that this drug combination would be beneficial in regards to treating metastatic HER2+ breast cancers. However, the pyrotinib group suffered more from adverse effects like diarrhea and hand-foot syndrome [40]. In the PHENIX trial, patients with CNS metastases treated with pyrotinib showed considerable improvement, and the incidence of new brain metastases in those without at baseline was drastically reduced when compared to the control group (1.2% versus 3.6%) [39]. However, pyrotinib was shown to be less effective in groups of people suffering from cancers with higher total number of mutations (TMB) [42]. Pyrotinib also showed a lower PFS rate in patients with liver metastases (8.7 months) when compared with patients without liver metastases (12.3 months) [41]. Overall, pyrotinib is regarded as a promising alternative drug therapy that, while is yet to be approved by the FDA, is already approved for use in China [39].
In conclusion, cytotoxic chemotherapy for breast cancer has made substantial progress in the last ten years, with several ground-breaking trials demonstrating a distinct survival benefit for newer treatments. The prognosis for patients with HER2-positive breast cancer changed substantially with the introduction of trastuzumab into standard therapy. Trastuzumab use; however, was still fraught with problems, including its use in low-risk or advanced disease, cardiovascular safety in geriatric patients, and drug resistance. Neratinib has been described as an orally active, tyrosine kinase inhibitor that irreversibly inhibits HER1 and HER2 receptors. Pyrotinib is the newest drug undergoing clinical trials as an anti-HER+ breast cancer chemotherapeutic agent, a second-generation, irreversible pan-HER tyrosine kinase receptor inhibitor which targets the EGFR, HER1, HER2 and HER4 receptors. These drugs have shown great promise and do not have the drawbacks trastuzumab poses. Immune checkpoint blockades, which have an astonishing clinical success rates and low safety profiles, have propelled themselves to the forefront of cancer treatment. Immunotherapy through immune checkpoint blockade produces long-lasting effects, improves survival rates in difficult-to-treat cancers and is changing the way that many neoplasms, like breast cancer, are treated. Positive outcomes have been seen with monotherapy or in combination with chemotherapy in the neo-adjuvant and metastatic settings. It is certain that more groundbreaking advances in this field would occur in the next decade.
Conflicts of Interest
These authors declare no conflicts of interest.
Author Contributions
All authors contributed equally to the conceptualization, project design, collection and assembly of data, writing and editing of the manuscript. All authors have read and agreed to the published version of the manuscript.
Funding
This research project received no external funding.
Acknowledgments
We wish to extend special thanks to Kelechukwu Ughagwu for proofreading the article and Adebayo Kehinde and Oderemi Oluwagbemisola for their inputs in the abstract and conclusions.
References
- HER-2 Positive Breast Cancer - a Mini-Review Asif HM , Sultana S, Ahmed S, Akhtar N, Tariq M. Asian Pacific journal of cancer prevention: APJCP.2016;17(4). CrossRef
- HER2. a 'predictive factor' ready to use in the daily management of breast cancer patients? Piccart MJ , Di Leo A, Hamilton A. European Journal of Cancer (Oxford, England: 1990).2000;36(14). CrossRef
- Her2-positive breast cancer: herceptin and beyond Dean-Colomb W, Esteva FJ . European Journal of Cancer (Oxford, England: 1990).2008;44(18). CrossRef
- The role of HER2 in cancer therapy and targeted drug delivery Tai W, Mahato R, Cheng K. Journal of Controlled Release: Official Journal of the Controlled Release Society.2010;146(3). CrossRef
- Human Epidermal Growth Factor Receptor 2 (HER2) in Cancers: Overexpression and Therapeutic Implications Iqbal N, Iqbal N. Molecular Biology International.2014;2014. CrossRef
- An overview of HER2 Lohrisch C, Piccart M. Seminars in Oncology.2001;28. CrossRef
- The oncogene HER2: its signaling and transforming functions and its role in human cancer pathogenesis Moasser MM . Oncogene.2007;26(45). CrossRef
- Prospect for Anti-HER2 Receptor Therapy in Breast Cancer Brand FX , Ravanel N, Gauchez AS , et al . Anticancer Res.2006;26(1B):463-470. Accessed September 3, 2022. https://ar.iiarjournals.org/content/26/1B/463.
- Mechanisms of receptor tyrosine kinase activation in cancer Du Z, Lovly CM . Molecular Cancer.2018;17(1). CrossRef
- Multiple roles of the PI3K/PKB (Akt) pathway in cell cycle progression Liang J, Slingerland JM . Cell Cycle (Georgetown, Tex.).2003;2(4).
- Using Clinical Proteomics to Discover Novel Anti-Cancer Targets for MAb Therapeutics. Advances in Cancer Management. Published online January 27, 2012 G. E, R. T . . CrossRef
- Progress in adjuvant chemotherapy for breast cancer: an overview Anampa J, Makower D, Sparano JA . BMC Medicine.2015;13(1). CrossRef
- Chemotherapy for advanced HER2-negative breast cancer: Can one algorithm fit all? Miglietta F, Dieci MV , Griguolo G, Guarneri V, Conte PF . Cancer Treatment Reviews.2017;60. CrossRef
- Long-term toxic effects of adjuvant chemotherapy in breast cancer Azim HA , Azambuja E, Colozza M, Bines J, Piccart MJ . Annals of Oncology: Official Journal of the European Society for Medical Oncology.2011;22(9). CrossRef
- Current challenges in HER2-positive breast cancer Puglisi F , Fontanella C , Amoroso V , et al . Crit Rev Oncol Hematol.2016;98. CrossRef
- HER2-amplified breast cancer: mechanisms of trastuzumab resistance and novel targeted therapies Gajria D, Chandarlapaty S. Expert Review of Anticancer Therapy.2011;11(2). CrossRef
- Immunotherapy of cancer Borghaei H, Smith MR , Campbell KS . European Journal of Pharmacology.2009;625(1-3). CrossRef
- Strategies for immunotherapy of cancer Melief CJ , Toes RE , Medema JP , Burg SH , Ossendorp F, Offringa R. Advances in Immunology.2000;75. CrossRef
- Checkpoint inhibitors in breast cancer - Current status Polk A, Svane I, Andersson M, Nielsen D. Cancer Treatment Reviews.2018;63. CrossRef
- Immune checkpoint inhibitors: Key trials and an emerging role in breast cancer Gaynor N, Crown J, Collins DM . Seminars in Cancer Biology.2022;79. CrossRef
- Targeting immune checkpoints in breast cancer: an update of early results Solinas C, Gombos A, Latifyan S, Piccart-Gebhart M, Kok M, Buisseret L. ESMO open.2017;2(5). CrossRef
- Immune Checkpoint Inhibitors Haanen JBAG , Robert C. Progress in Tumor Research.2015;42. CrossRef
- Immune Checkpoint Inhibitor Therapy: Key Principles When Educating Patients Wood LS , Moldawer NP , Lewis C. Clinical Journal of Oncology Nursing.2019;23(3). CrossRef
- Combination of Pertuzumab and Trastuzumab in the Treatment of HER2-Positive Early Breast Cancer: A Review of the Emerging Clinical Data Jagosky M, Tan AR . Breast Cancer (Dove Medical Press).2021;13. CrossRef
- Adoptive-cell-transfer therapy for the treatment of patients with cancer Dudley ME , Rosenberg SA . Nature Reviews. Cancer.2003;3(9). CrossRef
- Pembrolizumab monotherapy for previously treated metastatic triple-negative breast cancer: cohort A of the phase II KEYNOTE-086 study Adams S, Schmid P, Rugo HS , Winer EP , Loirat D, Awada A, Cescon DW , et al . Annals of Oncology: Official Journal of the European Society for Medical Oncology.2019;30(3). CrossRef
- From bench to bedside: the growing use of translational research in cancer medicine Goldblatt EM , Lee W. American Journal of Translational Research.2010;2(1).
- Emerging drugs targeting human epidermal growth factor receptor 2 (HER2) in the treatment of breast cancer Awada G, Gombos A, Aftimos P, Awada A. Expert Opinion on Emerging Drugs.2016;21(1). CrossRef
- Molecular Mechanisms and Translational Therapies for Human Epidermal Receptor 2 Positive Breast Cancer Lv Q, Meng Z, Yu Y, Jiang F, Guan D, Liang C, Zhou J, Lu A, Zhang G. International Journal of Molecular Sciences.2016;17(12). CrossRef
- Lapatinib for advanced or metastatic breast cancer Opdam FL , Guchelaar H, Beijnen JH , Schellens JHM . The Oncologist.2012;17(4). CrossRef
- Lapatinib, a Dual-Targeted Small Molecule Inhibitor of EGFR and HER2, in HER2-Amplified Breast Cancer: From Bench to Bedside Tsang RY , Sadeghi S, Finn RS . Clinical Medicine Insights: Therapeutics.2011;3. CrossRef
- The Clinical Efficacy and Safety of Neratinib in Combination with Capecitabine for the Treatment of Adult Patients with Advanced or Metastatic HER2-Positive Breast Cancer Chilà G, Guarini V, Galizia D, Geuna E, Montemurro F. Drug Design, Development and Therapy.2021;15. CrossRef
- Lapatinib with trastuzumab for HER2-positive early breast cancer (NeoALTTO): a randomised, open-label, multicentre, phase 3 trial Baselga J, Bradbury I, Eidtmann H, Di Cosimo S, Azambuja E, Aura C, Gómez H, et al . Lancet (London, England).2012;379(9816). CrossRef
- Neratinib in HER-2-positive breast cancer: results to date and clinical usefulness Chan A. Therapeutic Advances in Medical Oncology.2016;8(5). CrossRef
- Pyrotinib: a new promising targeted agent for human epidermal growth factor receptor 2-positive breast cancer Perachino M, Arecco L, Martelli V, Lambertini M. Translational Breast Cancer Research.2020;1(0). CrossRef
- Phase II study of neratinib in older adults with HER2 amplified or HER2/3 mutated metastatic breast cancer Yuan Y, Lee JS , Yost SE , Stiller T, Blanchard MS , Padam S, Katheria V, Kim H, et al . Journal of Geriatric Oncology.2021;12(5). CrossRef
- New Therapeutics in HER2-Positive Advanced Breast Cancer: Towards a Change in Clinical Practices?pi Mezni E, Vicier C, Guerin M, Sabatier R, Bertucci F, Gonçalves A. Cancers.2020;12(6). CrossRef
- Neratinib Plus Capecitabine Versus Lapatinib Plus Capecitabine in HER2-Positive Metastatic Breast Cancer Previously Treated With ≥ 2 HER2-Directed Regimens: Phase III NALA Trial Saura C, Oliveira M, Feng Y, Dai M, Chen S, Hurvitz SA , Kim S, et al . Journal of Clinical Oncology: Official Journal of the American Society of Clinical Oncology.2020;38(27). CrossRef
- Pyrotinib plus capecitabine versus lapatinib plus capecitabine for the treatment of HER2-positive metastatic breast cancer (PHOEBE): a multicentre, open-label, randomised, controlled, phase 3 trial Xu B, Yan M, Ma F, Hu X, Feng J, Ouyang Q, Tong Z, et al . The Lancet. Oncology.2021;22(3). CrossRef
- Pyrotinib in the Treatment of Women With HER2-Positive Advanced Breast Cancer: A Multicenter, Prospective, Real-World Study Zhang L, Wu X, Zhou J, Zhu M, Yu H, Zhang Y, Zhao Y, Han Z, et al . Frontiers in Oncology.2021;11. CrossRef
- Pyrotinib or Lapatinib Combined With Capecitabine in HER2-Positive Metastatic Breast Cancer With Prior Taxanes, Anthracyclines, and/or Trastuzumab: A Randomized, Phase II Study Ma F, Ouyang Q, Li W, Jiang Z, Tong Z, Liu Y, Li H, et al . Journal of Clinical Oncology: Official Journal of the American Society of Clinical Oncology.2019;37(29). CrossRef
- Effectiveness and safety of pyrotinib-based therapy in patients with HER2-positive metastatic breast cancer: A real-world retrospective study Li C, Bian X, Liu Z, Wang X, Song X, Zhao W, Liu Y, Yu Z. Cancer Medicine.2021;10(23). CrossRef
- Trastuzumab | 180288-69-1. (n.d.). Retrieved November 7, 2023, from https:// www.chemicalbook.com/ ChemicalProductProperty_EN_CB61074602.htm .
- Role of lapatinib in the first-line treatment of patients with metastatic breast cancer Oakman Ca, Pestrin M, Zafarana E, Cantisani E, Di Leo A. Cancer Management and Research.2010;2.
- Study of Interactions of an Anticancer Drug Neratinib With Bovine Serum Albumin: Spectroscopic and Molecular Docking Approach Wani T. A , Bakheit A. H , Abounassif M. A , & Zargar S. Frontiers in Chemistry.2018;6. CrossRef
- Pyrotinib (SHR-1258) | EGFR/HER2 Inhibitor | MedChemExpress. (n.d.). Retrieved November 7, 2023, from https://www.medchemexpress.com/Pyrotinib.html .
License

This work is licensed under a Creative Commons Attribution-NonCommercial 4.0 International License.
Copyright
© Asian Pacific Journal of Cancer Biology , 2024
Author Details